Quantum Technologies and Quantum Computing in Russia
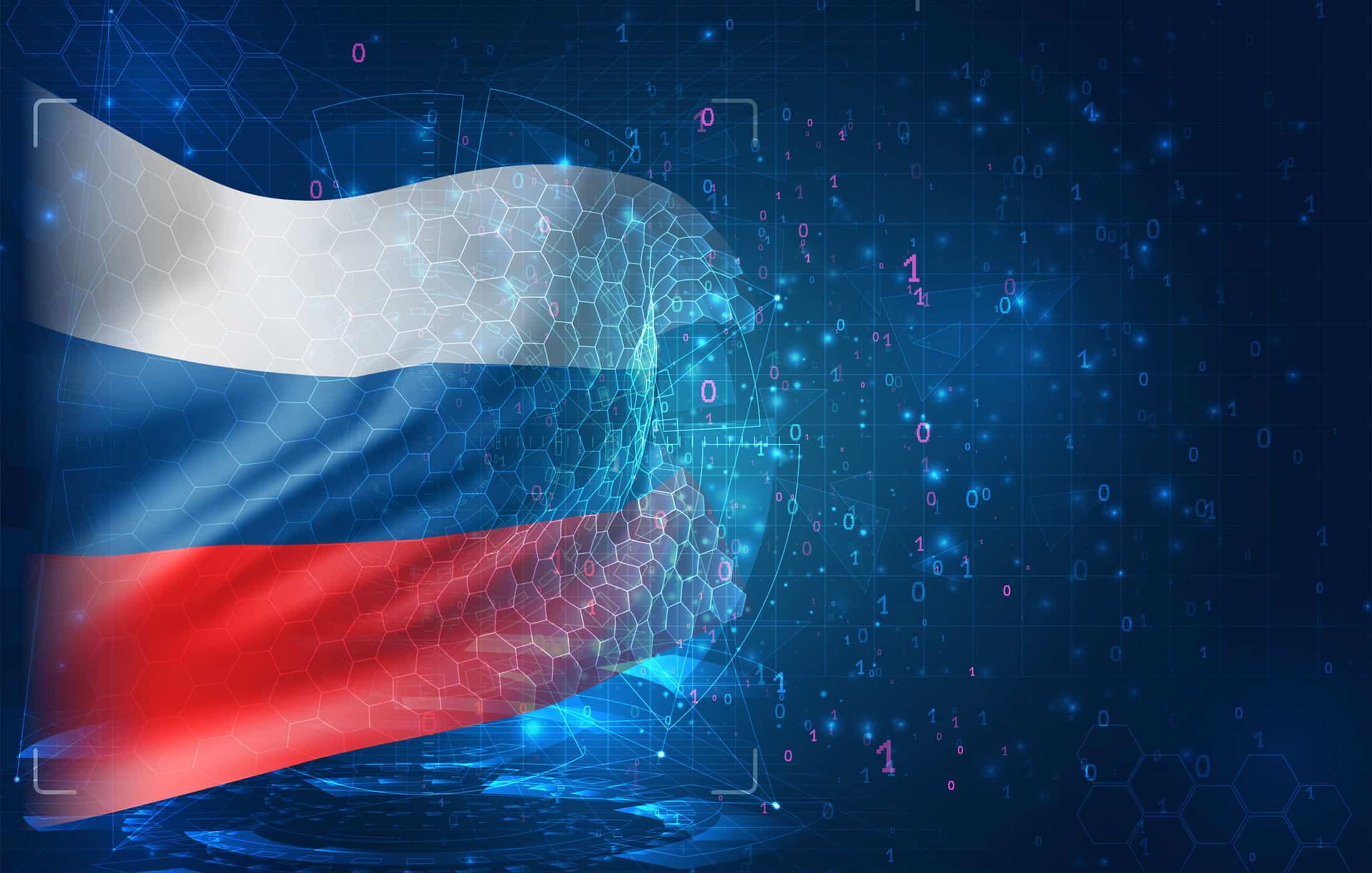
Table of Contents
Historical Context of Russia’s Quantum Research
Russia’s engagement with quantum science dates back to the Soviet era, which produced a strong foundation of theoretical physics and early quantum experiments. This legacy endures in the modern era – Russian experts often note that the “Soviet school of quantum physics was one of the best in the world,” providing a deep talent pool for today’s initiatives. In the 2010s, Russia began explicitly organizing its quantum research efforts. A key milestone was the establishment of the Russian Quantum Center (RQC) in 2010 at the Skolkovo innovation hub as a private research institution focused on fundamental and applied quantum physics. RQC quickly garnered support, securing over 2 billion rubles (~€30 million) in funding from competitive grants and private investors like Gazprombank. This signaled a public-private interest in keeping pace with the “second quantum revolution.” Soon after, regional centers emerged (e.g. a quantum center in Kazan in 2014) and Russian universities expanded quantum research programs.
By the late 2010s, the government elevated quantum technology as a strategic priority. Quantum tech was included among the “strategically important cross-cutting directions” of national programs such as the National Technology Initiative (NTI) and the Digital Economy National Program. In 2017–2018, two specialized NTI centers were created: the Quantum Technology Center at Lomonosov Moscow State University (MSU) and an NTI Center for Quantum Communications at the National University of Science and Technology MISiS. Each received roughly 2 billion rubles (~€30 million) over five years from the Ministry of Science and Higher Education and the Russian Venture Company, aimed at building quantum devices and educational programs. These early efforts culminated in a Russian Quantum Technologies Roadmap adopted around 2019 as part of the national “Digital Economy” program. The roadmap laid out goals in four areas – quantum computing, quantum communications, quantum metrology and sensing, and enabling technologies – providing a coordinated strategy through 2024. Importantly, three major state-owned enterprises stepped up to lead each domain: Rosatom (the State Atomic Energy Corporation) took charge of quantum computing, Russian Railways (RZhD) took charge of quantum communications, and Rostec (the state defense tech conglomerate) assumed leadership in quantum sensing and metrology. This clear delegation, backed by high-level political support, set the stage for an accelerated push in quantum technologies in Russia.
Quantum Computing: Current State and Advancements
Government Initiatives and National Quantum Program
Russia’s quantum computing program is driven largely by government initiatives and roadmaps. Rosatom, as the lead agency for quantum computing, launched a national program in late 2019 dedicated to developing practical quantum computers and algorithms. In 2020, Rosatom announced an investment of roughly $790 million to fund quantum computing R&D across Russia. A centerpiece of this effort was the creation of the National Quantum Laboratory (NQL), a consortium to unite leading labs and companies. Launched as a federal project in late 2020, the NQL brings together institutions such as MISiS, MIPT (Moscow Institute of Physics and Technology), MSU, the Lebedev Physical Institute of the Russian Academy of Sciences (RAS), RQC, and the Skolkovo Foundation. The NQL is headed by Ruslan Yunusov – a co-founder of RQC and now head of Rosatom’s quantum project office – and aims to pool expertise, build new infrastructure, and train talent in quantum computing. Construction is underway on a 2,000 m² nanofabrication center and a 3,500 m² advanced laboratory complex at Skolkovo to provide cutting-edge equipment for NQL researchers. The consortium’s mandate includes developing quantum processors across four main platforms – superconducting circuits, trapped ions, neutral atoms, and photonics – reflecting a broad approach similar to other nations. This multiplatform strategy is meant to “identify the most efficient and accurate systems for various tasks” by comparing their performance. The national quantum roadmap set an initial goal of creating a 30–100 qubit quantum computer by 2024, with an eventual aim for a universal machine of several hundred qubits that could tackle economically relevant problems.
Progress so far suggests Russia is on track with these milestones. Early prototypes were modest – just a 2-qubit device a few years ago – but by 2021–2022 teams had built 4- and 8-qubit superconducting processors in the lab. In July 2023, Rosatom announced a 16-qubit quantum computer based on trapped ions, which was showcased to President Vladimir Putin as a proof-of-concept system. This device, demonstrated at the “Future Technologies Forum” in Moscow, uses ytterbium ions as qubits and highlights Russia’s interest in ion trap approaches. Rosatom’s press releases noted that the effort stemmed from a decision in 2019 to include ion traps in the national roadmap and that scientists “lived in the laboratory for over three years” to achieve the 16-qubit prototype. By late 2024, Russia hit its roadmap’s headline target: 50-qubit quantum computers were realized on multiple platforms. Rosatom’s CEO Alexei Likhachev reported to Putin that a 50-qubit ion-based quantum computer had been completed. Around the same time, a separate team from MSU and RQC unveiled a 50-qubit neutral rubidium atom quantum computer prototype in December 2024. This neutral-atom device was developed under the national roadmap and delivered on the promise to reach 50 qubits before 2025. (Notably, RQC clarified that it now has two 50-qubit systems: one ionic quantum computer demonstrated in September 2024, and a second prototype using neutral atoms unveiled in December.) With these achievements, Russia has joined the select group of nations capable of building devices at the 50+ qubit scale – a “psychologically important mark” that signals competitiveness in the global quantum race.
Looking forward, Russia’s quantum computing roadmap to 2030 foresees steady improvements in scale and performance. The next step is to build a ~75-qubit quantum computer by 2025, pushing closer to the threshold where quantum hardware could outperform classical supercomputers on certain tasks. However, the emphasis is not only on qubit count but also qubit quality. Russian researchers recognize that to solve useful problems, qubits must have very high fidelity (operation accuracies on the order of 99.7–99.9%) and error rates well below 1%. Thus, a major R&D focus is on improving coherence and gate accuracy through better materials and designs. In Yunusov’s words, “the error rate should be unambiguously less than 1%, and ideally 0.1–0.3%, to actively deal with errors and get good results.” Achieving this will require breakthroughs in materials science – a point Russian experts highlight as crucial for “technological sovereignty.” Upcoming efforts (e.g. at the Future Technology Forum) are targeting the development of new materials with atomic-level precision for quantum chips, since “the possibilities of silicon are almost exhausted” for quantum needs. In parallel, Russia is exploring novel qubit implementations (sometimes termed “nuclear” or alternative platforms in press statements) – these could refer to qubits based on nuclear spins or molecular systems, indicating an openness to unconventional technologies beyond the main four platforms. Overall, backed by substantial federal funding and coordination, Russia’s quantum computing initiative has rapidly grown from laboratory experiments to multi-qubit prototypes. The state-led approach – building a full stack from education to fabrication – is intended to ensure Russia can develop a universal quantum computer by the end of the decade and apply it to practical industrial and scientific problems.
Leading Research Institutions and Collaborators
A network of research institutions underpins Russia’s quantum computing progress. Many of these are part of the National Quantum Laboratory or related consortia, each contributing expertise:
Russian Quantum Center (RQC) – Founded in 2010 at Skolkovo, RQC has been a driving force in Russia’s quantum effort. It has attracted young scientists (including Russian expatriates) and secured significant private funding to run multiple labs. RQC conducts research across quantum computing (both theory and experiment), quantum communications, and basic quantum science. It also spins off startups (e.g. QRate) and collaborates with government and industry. Co-founded by leading physicists, RQC serves as a bridge between academia and industry needs.
Lomonosov Moscow State University (MSU) – Home to the Quantum Technology Center (created under NTI), MSU engages in quantum hardware and theory research. MSU physicists have been central to projects like the 50-qubit neutral atom computer and advanced quantum sensors. MSU’s long tradition in quantum optics and its International Laser Center also support quantum communication experiments.
National University of Science and Technology MISiS – MISiS in Moscow hosts the NTI Center for Quantum Communications and one of the first labs on superconducting qubits (established 2011). MISiS researchers helped draft the national quantum roadmap in 2019. The university is active in superconducting quantum processor development, quantum materials, and photonics. MISiS also collaborates on multi-node quantum network testbeds (as seen in its interuniversity network project).
Moscow Institute of Physics and Technology (MIPT) – MIPT (Phystech) established a superconducting qubit lab in 2014 and contributes to quantum algorithm and software research. It was part of a three-year joint project (with MISiS) supported by the Foundation for Advanced Research (FPI, a DARPA-like agency) and Rosatom to develop superconducting quantum computing elements. MIPT’s theoretical groups also explore quantum information theory and error correction.
RAS Institutes – Several institutes of the Russian Academy of Sciences play key roles. The P.N. Lebedev Physical Institute (LPI) in Moscow is involved in quantum computing (especially optical and atomic clocks) and quantum sensing projects. For example, LPI leads development of a compact ytterbium-ion optical clock for the GLONASS satellite system (in partnership with Skoltech and industry). The RAS Institute of Laser Physics in Novosibirsk works on cold-ion/atom control (part of the clock project). The RAS Institute of Solid State Physics and others support materials research for qubits. Having RAS onboard brings in fundamental science expertise and lab infrastructure across the country.
Skolkovo Institute of Science and Technology (Skoltech) – Skoltech (in Moscow) collaborates closely with RQC and others. It has groups focusing on quantum algorithms, photonic quantum computing, and quantum optics. Skoltech participated in the optical clock project and hosts a “Deep Quantum Lab” working on variational quantum algorithms and superconducting circuit theory. International faculty hires via megagrants (e.g. Prof. Jacob Biamonte in quantum algorithms) have bolstered Skoltech’s profile.
ITMO University (St. Petersburg) – ITMO is renowned for photonics and was selected to host a National Center for Quantum Internet in 2019. ITMO’s researchers work on quantum communications protocols, quantum networking (e.g. developing trusted node networks and entanglement swapping techniques), as well as nanophotonic devices for quantum light manipulation. It also offers specialized master’s programs in quantum technologies. Saint Petersburg’s strong optics community (including teams at St. Petersburg State University) contributes to quantum cryptography and sensing research as well.
Regional Centers – Outside Moscow/Petersburg, centers like the Kazan Quantum Center (at Kazan National Research Technical University) focus on areas such as quantum optics, quantum memory, and spectroscopy. Novosibirsk State University and Novosibirsk State Technical University launched a quantum research lab in Siberia (noted in early superconducting qubit projects). These broaden the geographic spread of expertise.
This ecosystem is reinforced by educational initiatives and talent programs. NQL, for instance, is implementing quantum education curricula from high school onwards and creating new standards for training quantum engineers. The goal is to cultivate a “new generation of technologists” skilled in both research and commercialization. Programs at universities like MIPT’s Department of Technological Business (focused on tech entrepreneurship) are grooming graduates who can translate quantum R&D into industry solutions. Russia has also leveraged its Megagrant program to recruit internationally renowned scientists to set up labs in Russia with €3–5 million grants. Dozens of top quantum scientists (Russian and foreign) have participated, helping to jump-start research in areas from quantum computing to metrology.
Private Sector and Startup Developments
In Russia’s quantum landscape, the public sector and academia are closely intertwined with emerging private companies. Unlike in the U.S., where big corporations lead much of the quantum R&D, in Russia the state-driven program is the primary engine – but it has spawned startups and corporate R&D projects responding to national goals. A prime example is QRate, a tech company specializing in quantum communications hardware. QRate was spun off from RQC and has developed fiber-optic quantum key distribution (QKD) systems that are now approaching commercial readiness. In 2024, a Russian-led team reported that QRate’s QKD system passed extensive security tests and was “ready for formal certification” under national cryptographic standards. This is a crucial step before wide deployment, ensuring the system is robust against known attacks (for instance, tests identified detector vulnerabilities and implemented countermeasures to foil potential eavesdroppers). Such progress by a startup underscores how government-backed research (RQC’s QKD prototypes) has moved toward real-world products for secure communication.
Another notable company is Terra Quantum, which, while headquartered in Switzerland, has deep Russian roots (founded by Russian physicists and entrepreneurs) and R&D ties in Russia. Terra Quantum works on quantum algorithms, hybrid quantum-classical computing, and quantum cryptography services. It gained attention for claiming breakthroughs in quantum encryption (though those claims met skepticism). Amid geopolitical tensions, Terra Quantum reportedly minimized its overt Russian affiliations, but the company’s existence reflects the global aspirations of Russian quantum talent. It serves international clients with “Quantum-as-a-Service” offerings and exemplifies how Russian experts are also operating on the world stage via private ventures.
Within Russia, large tech-oriented corporations and banks have started their own quantum exploratory projects, often in collaboration with the aforementioned research institutes. Gazprombank, for example, funded a research initiative in quantum machine learning with an annual budget of about €1.4 million, partnering with RQC to investigate how quantum algorithms could improve financial services. Sberbank, the country’s largest bank, and other financial institutions have also participated in quantum network pilots (Sberbank took part in the QKD demonstration at SPIEF 2019, see below) and are interested in quantum computing for complex portfolio optimization and risk modeling. Rostelecom, the national telecom operator, is involved in quantum communication infrastructure projects (as detailed in the next section). Meanwhile, defense-industrial companies under Rostec are likely investing in quantum sensing (though details are often not public). The startup ecosystem is expected to grow under the roadmap’s vision – the 2019 Quantum Technologies roadmap predicted that by 2024 Russia should see “several dozen startups” in quantum tech able to compete globally. Indeed, small companies such as KuSpace Tech have emerged; KuSpace partnered with MISIS and RQC on satellite QKD experiments (providing a ground station for a quantum satellite link).
This interplay of state, academia, and startups is by design. As Rosatom’s leadership noted, the consolidation of efforts under a single “brand” (like NQL) and sharing of knowledge between big players and nimble startups is seen as the way to propel Russia’s standing on the global stage. The NQL explicitly encourages small startup teams to join and promises them support, incubators, and access to funding. The goal is to create an ecosystem where fundamental research leads to prototypes, which then spawn companies that bring products (be it quantum computers-on-the-cloud, QKD devices, or specialized sensors) to the market. While Russia’s private sector in quantum is still nascent compared to the U.S. or EU, these initiatives show a clear recognition that commercialization and innovation by companies will be crucial to fully exploit quantum technology.
Quantum Communications and Cryptography
Quantum communication is a major focus of Russia’s quantum strategy, with the twin goals of developing quantum-secure networks and protecting against the threat of quantum code-breaking. Under the national roadmap, quantum communications is one of the “three key focuses” (alongside computing and sensing) and is led by Russian Railways in partnership with telecom companies. Significant progress has been made in both infrastructure deployment and technology development for quantum cryptography.
Quantum Key Distribution Networks
Russia is actively building out quantum key distribution networks to secure data links for government and industry. A flagship project has been the creation of a Moscow–St. Petersburg quantum communication backbone, spanning roughly 700–800 km. This was first provisioned in 2021 by TransTelekom (a subsidiary of Russian Railways) in cooperation with Rostelecom, making it the first long-distance quantum-secured fiber link in Russia. By April 2021, TTK announced the completion of this Moscow–Petersburg QKD network, which essentially forms a trunk line capable of carrying encrypted traffic secured by quantum keys between Russia’s two biggest cities. This implementation aligns with the national quantum communications roadmap, which calls for 7,000 km of quantum networks by end of 2024. The plan entails extending QKD-enabled fiber routes across major cities and industrial hubs. Indeed, as of late 2021, besides the Moscow–Petersburg trunk, quantum-secured links were operating in several scientific, financial, and commercial institutions on a smaller scale. For example, MISIS and the Moscow Technical University of Communications and Informatics (MTUCI) built and tested a five-node quantum network linking their campus labs in Moscow. Such pilot networks allow researchers to experiment with routing quantum keys through multiple nodes and to integrate QKD with existing classical infrastructure.
A showcase of Russia’s quantum communications capabilities occurred at the St. Petersburg International Economic Forum (SPIEF) in June 2019. There, RQC and its partners demonstrated a live quantum-encrypted video conference between the booths of Gazprombank, Sberbank, and PwC. The demonstration used RQC/QRate QKD devices to exchange encryption keys, which then secured a video call in real-time. This event – supported by the Roscongress Foundation and noted by government advisors – was intended to signal that Russia could deploy quantum-secure communication in practical settings like banking. The Presidential adviser Anton Kobyakov highlighted at SPIEF that “according to the roadmap, Russia should close the gap with world leaders in quantum computing and reach the global level in quantum communications by 2024”. He also stated an ambition for Russian companies to capture at least 8% of the global quantum communications market – including exporting technology to CIS, Southeast Asia, Latin America, and BRICS countries. This was an early articulation of Russia’s intent not just to use quantum tech domestically but also to be an international provider of quantum-secure systems. The SPIEF demo also saw Rostelecom present a working concept of a multi-node quantum network, indicating that the largest telecom operator was actively engaged in the field. RQC’s CEO at the time, Ruslan Yunusov, noted that this joint demonstration “shows our readiness to create a full-fledged nationwide quantum key distribution network that all market players can use.”
Since then, concrete steps have been taken: by late 2024, according to officials, over 5,000 km of quantum fiber links were either deployed or in development, heading toward the 7,000 km goal. Focus areas include connecting governmental sites (to secure sensitive communications between ministries), financial institutions (to secure interbank data links), and critical infrastructure like power grids. The fact that Russian Railways is a lead player is notable – the railway owns vast fiber-optic networks along its tracks, which can be leveraged for QKD without needing to lay much new fiber. The involvement of Rostelecom and other telecom carriers means the quantum network can piggyback on existing telecom infrastructure, inserting quantum channels where needed. However, distance limitations of ground-based QKD (typically tens of kilometers without trust nodes, due to photon losses) mean that to go truly long-range (hundreds or thousands of km) either many trusted repeater nodes are required or satellites must be used. Russia is exploring both. The terrestrial strategy uses intermediate trusted nodes every 50–100 km or so (secure relay stations) – e.g. the Moscow–Petersburg QKD line likely has such nodes. For the global scale, Russia has turned to satellite QKD in collaboration with China, as described later in the Global Position section (China’s Micius quantum satellite was used in 2023 to exchange quantum-secured data between Moscow and Urumqi). Eventually, Russia may launch its own quantum communication satellites as well, to have an independent quantum-encrypted satellite link for governmental use.
Quantum Cryptography and Security Efforts
In tandem with building QKD networks, Russia is advancing the underlying quantum cryptographic technologies. QRate’s progress toward a certified QKD system is one example: their device implements the standard BB84 protocol with decoy states over optical fiber and has been hardened against known attacks (like detector blinding) by adding monitoring of detector photocurrent, etc. Once certified by Russia’s Federal Security Service (FSB) or related authorities, such QKD systems can be deployed in high-security environments (government communications, bank data centers, etc.) with the confidence that they meet national cryptographic requirements. It’s expected that domestic QKD devices will be integrated into existing encryption infrastructure – for instance, to frequently re-key VPNs or encryptors that secure Russia’s critical networks. Government interest in QKD is motivated by the desire for “unprecedented level of security” in communications. Quantum keys, unlike classical key exchange, offer provable eavesdrop detection – any attempt to intercept the key will disturb the quantum states and be noticed. This is attractive for protecting everything from military communications to everyday civilian data (as mentioned by a MISIS press release, even something like transmitting credit card details could be made secure against future quantum decryption by using QKD-provided keys).
Beyond QKD, Russia is cognizant of the looming threat that quantum computing poses to classical cryptography. Today’s common encryption algorithms (RSA, Diffie–Hellman, elliptic curve cryptography) could be broken by a sufficiently powerful quantum computer running Shor’s algorithm. Russian researchers have noted that what might take a classical supercomputer “millions of years” to brute-force could take a quantum computer mere “hours” once large-scale quantum machines are operational. This scenario – where encrypted data (for example, government secrets or personal data) can be retroactively decrypted – is seen as a serious vulnerability. Therefore, in parallel to quantum communications, Russia is investing in quantum-resistant (post-quantum) cryptography (PQC). Efforts here are somewhat less publicly visible but are underway in cryptographic research labs and institutions. According to an overview by analysts, Russia (like China) is expected to adopt “slightly different” PQC standards from those of the U.S., likely selecting algorithms based on similar hard mathematical problems but vetted independently. In practice, this means Russian cryptographers are evaluating the finalists from the NIST PQC competition (lattice-based schemes, hash-based signatures, etc.) and also developing indigenous PQC algorithms that could replace or augment Russia’s GOST encryption standards. For instance, Russian scientists have explored post-quantum digital signature schemes using the Streebog hash (Russia’s standard hash function), and academic groups are testing lattice-based encryption performance on domestic hardware. While as of 2022 no official Russian PQC standard had been announced (understandable, since global PQC standards were only just finalized by NIST in 2022–2023), the field is “focused on R&D activities to develop such algorithms, verify existing solutions and test concepts and pilots.” The lack of “unified and battle-proven” PQC standards is acknowledged, which is why Russian experts stress carefully selecting optimal algorithms and preparing for a transition before quantum computers mature.
In summary, Russia’s approach to securing information in the quantum era is two-pronged: deploy quantum cryptography (QKD) for high-value links now (ensuring forward security even against future quantum adversaries), and migrate to post-quantum classical algorithms for broader use (so that public-key infrastructures and encryption used in day-to-day communications remain safe). Government communications systems are likely already experimenting with combined solutions – e.g. using QKD keys in one layer and PQC algorithms in another as a defense-in-depth. The geopolitical dimension cannot be ignored: Russian officials see quantum-secure communication as vital for national sovereignty, especially given global surveillance capabilities. By investing in indigenous QKD and PQC, Russia aims to avoid reliance on foreign encryption that could be compromised. This is highlighted by statements that quantum tech will secure data “in geopolitical contexts.” Furthermore, Russia’s participation in international quantum communication collaborations (with China, and potentially with partners in BRICS) suggests an intent to create a quantum-secure communications bloc independent of Western-controlled networks. In essence, quantum communications in Russia is not just a scientific endeavor but a strategic infrastructure project to “secure national infrastructure against quantum decryption threats.”
Quantum Sensing and Metrology Developments
Quantum sensing – the use of quantum phenomena to achieve ultra-sensitive measurements of physical quantities – is the third pillar of Russia’s quantum initiative (with Rostec as the lead). Applications range from defense and security to fundamental science and industry. Russia’s roadmap explicitly lists a variety of quantum sensor targets, including next-generation atomic clocks, inertial sensors, magnetometers, and imaging techniques. Russian teams are leveraging quantum effects (superposition, entanglement, quantum interference) to attain sensitivities far beyond classical device limits, which can confer strategic advantages.
One high-priority area is quantum timing and navigation. In the 2010s, Russia initiated a project to develop a compact optical atomic clock that could be deployed in its GLONASS satellite navigation system (GLONASS is Russia’s analog to GPS). By 2019, important results had been achieved: a consortium including the Lebedev Institute (RAS), Skoltech, the Institute of Laser Physics in Novosibirsk, and companies like Avesta and Russian Space Systems had a working prototype optical clock based on a single trapped ytterbium ion. The clock is designed to fit in about a 1 m³ package and serve as a highly stable frequency standard. This effort, supported by the Federal Space Agency and the Ministry of Science, is aimed at eventually installing such quantum clocks on GLONASS satellites to dramatically improve their timekeeping (and thus positioning accuracy). A more precise GLONASS means better guidance for civilian and military users and greater independence from other navigation systems. Quantum clocks are also being pursued for ground applications – for instance, portable atomic clocks could synchronize telecommunications or serve as backup timing if GNSS signals are lost. The roadmap lists “optical atomic clocks” first among quantum sensor directions, underlining their importance.
Closely related are quantum inertial sensors. Recognizing that GPS/GNSS signals can be jammed or denied (something both Russia and other militaries actively plan for ), the development of ultra-accurate inertial navigation systems is crucial. Russia’s roadmap includes “gravimeters, accelerometers, and gyroscopes based on cold atoms” as well as “gyroscopes based on solid-state spin ensembles.” Cold-atom interferometer sensors use free-falling atoms in vacuum as a perfect reference, measured via matter-wave interference to sense accelerations or rotations with extreme precision. A quantum accelerometer or gyroscope could allow a submarine, aircraft, or spacecraft to navigate with high accuracy for long periods without external signals – essentially a quantum IMU (Inertial Measurement Unit). Both Russia and China have been investing in this as a way to mitigate GPS vulnerability on the battlefield. Though specific Russian prototypes are not public, research groups (likely at institutes like Ioffe or in collaboration with FPI) are working on integrating cold-atom sensors. Another approach listed is using solid-state spins (perhaps NV centers or other defects) in a gyroscope arrangement. Rostec entities (which include avionics and guidance system manufacturers) are presumably interested in transitioning these sensors to real platforms once mature. The advantage is significant: a quantum inertial navigator could provide positioning updates with minimal drift, enabling, for example, a missile to stay on target even if GPS is jammed, or an aircraft to land safely in electronic warfare conditions. National defense literature frequently cites quantum inertial navigation as a disruptive tech for the military.
In the realm of detection and imaging, quantum magnetometry is a standout capability being developed. Russian researchers, in collaboration with international partners, have been pushing the frontier of magnetometers using nitrogen-vacancy (NV) color centers in diamond. NV centers are atomic-scale defects in diamond that can be manipulated and read out via quantum techniques at room temperature. They are extremely sensitive to magnetic fields, electric fields, temperature, and strain. A joint project involving RQC, MSU’s Physics Department and Laser Center, Texas A&M University, the Lebedev Institute, the Kazan Quantum Center, and others has been developing high-resolution nano-scale thermometry and magnetometry techniques using NV centers. Supported by a megagrant and RFBR funds, this interdisciplinary effort has demonstrated that NV electron spins can be used to detect weak magnetic fields with unprecedented spatial resolution (on the order of nanometers). Essentially, they created fiber-coupled NV sensor probes that achieve nanoscale magnetic imaging and temperature mapping at the single-cell level. The sensitivity is so high that it opens new possibilities in fields from neuroscience (imaging neural activity via tiny magnetic signals) to materials science and even geology. With NV magnetometers, one could detect minuscule magnetic anomalies – potentially useful for locating submarines (which disturb Earth’s magnetic field slightly) or finding mineral deposits underground. In fact, the project highlights that such quantum magnetometers could measure weak fields relevant to “astrophysics, geosciences, and fundamental physics symmetries” as well as life sciences. For practical deployment, the integration of these sensors into fiber-optic probes is key, and the team demonstrated a scanned fiber probe with an NV sensor that can image magnetic fields in real time. This kind of technology could be adapted for defense (e.g., a drone equipped with a quantum magnetometer scanning for hidden vehicles or weapons) or for security (detecting magnetic signatures of electronic devices). Rostec companies in the optics and instrumentation sector (like Shvabe) would likely commercialize such devices for both civilian and military markets once ready.
Other quantum sensing initiatives in Russia include:
Quantum gravimetry: Using atom interferometry to measure gravity gradients. This can detect underground structures (tunnels, bunkers) or help in oil and gas exploration by sensing density anomalies. The roadmap’s mention of cold-atom gravimeters suggests ongoing projects.
Plasmonic and optical sensors: The roadmap also lists “plasmonic biosensors” and advanced photodetectors. Quantum plasmonic sensors could improve detection of biomolecules, toxins, etc., with implications for healthcare and security. Meanwhile, Russia has a legacy in optical metrology – e.g., Prof. D. N. Klyshko’s pioneering work on absolute calibration of single-photon detectors is carried forward in projects on quantum imaging and spectroscopy. For instance, using correlated photon pairs from parametric down-conversion, Russian scientists have developed new spectroscopy techniques (measuring IR absorption using visible detectors via quantum correlations). Such techniques can be applied to remote sensing or detecting substances at a distance with quantum illumination. There is even exploration of extending these methods into the THz range for potentially sensing concealed objects (THz waves can penetrate certain materials).
By placing Rostec in charge of quantum sensing, the government ensured that the defense sector guides these developments. The military applications are clear: precision navigation (quantum IMUs), timing (clocks), detection (magnetometers, gravimeters), and communication (quantum RF sensors) can all confer tactical advantages. For example, a stealth aircraft might be detectable by a sensitive quantum radar or magnetometer that notices the disturbance it makes in the ambient field, even if it evades conventional radar. Quantum sensors could also improve signal intelligence, by detecting faint communications or GPS spoofing attempts. The Arctic Institute recently highlighted the need for new quantum sensors to monitor undersea infrastructure in challenging environments, which aligns with Russian interests in securing Arctic undersea cables and detecting foreign submarines. In metrology, Russia’s investment in quantum standards (like optical clocks) also underpins its scientific research – precise measurements allow cutting-edge experiments (e.g., testing fundamental physical constants or enabling better synchronization in large telescopes or particle detectors).
In summary, Russia’s work in quantum sensing and metrology is broad and ambitious: from all-optical nanoscale sensors that can probe living cells, to strategic navigation systems that ensure resilience against electronic warfare. If quantum computers and communications are about information processing and security, quantum sensors are about mastering the physical environment with quantum-enhanced data. Russia sees this as a domain where it can capitalize on its rich physics tradition (many Nobel-worthy experiments in optics and metrology came from Russian scientists) and where relatively small devices can have outsized impact. By 2024, the roadmap aimed to have prototypes or even deployed units in many of these categories, and indeed some (clocks, NV sensors) have reached prototype stage. The coming years may see Russia integrate these quantum sensors into both civil infrastructure (e.g., for geological surveying, time distribution, medical imaging) and military platforms – quietly boosting capabilities in ways less publicly visible than a quantum computer, but highly potent.
Russia’s Global Position in Quantum Technology
On the international stage, Russia is positioning itself as a serious contender in the quantum technology race, though it faces stiff competition from the U.S., China, and the EU. By late 2024, Russian officials could claim that the country has joined the “select group of nations” with cutting-edge quantum achievements like 50-qubit processors. Indeed, developing multiple 50-qubit quantum computers (ion-based and neutral-atom) places Russia in a club that, aside from itself, only includes a few countries (the United States and China, primarily, with the EU and Canada making strides via companies and consortia). Ruslan Yunusov stated that Russia “has positioned itself among the global leaders in quantum computing, achieving significant milestones despite external challenges.” While some caution is warranted – for example, Russia’s 16-qubit ion computer was an annealing-type or specialized device, not yet a universal gate quantum computer on par with IBM’s 127-qubit processors – the rapid progress from 2 to 50 qubits in a short span is impressive. It indicates a narrowing of the gap with world leaders. Moreover, Russia’s strategy of pursuing multiple qubit platforms in parallel (superconducting, ionic, photonic, atomic) is similar to approaches in the U.S. and China, and increases the chance of finding a winning technology. As Yunusov explained, different platforms have different strengths and it’s wise to explore them all for various tasks. This breadth is a strength; for instance, Europe’s quantum efforts are sometimes spread across nations, whereas Russia can coordinate multiple approaches under one program.
Russia’s strengths in the global quantum arena include:
Strong Fundamental Science Base: Decades of excellence in physics and math give Russia a talent advantage. Its young scientists are “world-class specialists” grounded in a top-tier Soviet-era education legacy. This has enabled Russia to “leverage its Soviet-era expertise” to catch up in new fields. We see this in areas like quantum algorithms and theory – Russian researchers contribute to global quantum information science (for example, the concept of quantum suprematism in algorithms was formulated by RQC/Skoltech teams).
Coordinated National Strategy: The high-level political support for quantum tech in Russia (with President Putin being briefed on progress, and Rosatom’s director calling it a competition of “world scientific schools and technological giants” ) means initiatives get the backing they need. Russia formed a national program early (2019), comparable to the U.S. National Quantum Initiative (2018) and EU Quantum Flagship (2018). This led to consolidation of efforts (NQL) and clear allocation of responsibilities. Russian leaders explicitly modeled this on the experience of “American, Asian and European colleagues,” understanding that acting as a unified “single brand” helps on the global stage. Such focus prevents duplication and maximizes resource use – crucial given Russia’s budget, which while large, is still lower than U.S./China outlays.
Public-Private Synergy: Russia’s approach marries state funding with private involvement (banks, telecoms, startups). Institutions like Sberbank, Gazprombank, Rostelecom, etc., not only lobby for quantum tech (as future users) but also invest in pilot projects. This ensures that the research directions align with real-world needs (e.g., finance, secure communications) and that when technology matures, there are ready adopters domestically. It also helps create a nascent market and demand for quantum solutions within Russia, which is vital for sustaining progress.
Niche Expertise: In some sub-fields, Russia holds particular expertise that gives it an edge. For example, quantum communications is one area where Russia has achieved parity with leaders: it has operational metropolitan QKD networks and is working on satellite QKD with China. In fact, in January 2024 Russian and Chinese scientists demonstrated quantum-encrypted communication over 3,800 km – sending secure keys and images between a station near Moscow and one near Urumqi via China’s Mozi satellite. This successful test (sending an image encrypted with quantum keys between continents) was touted as evidence that a quantum communication network among BRICS nations is feasible. Russian researchers like Alexey Fedorov (MISIS/RQC) played key roles in this, showing Russia’s willingness to collaborate internationally in quantum projects that can extend its reach. Such cooperation with China is a strategic asset – effectively pooling expertise and infrastructure. Another niche is quantum metrology (optical clocks, etc.) where Russian labs (e.g., Lebedev Institute) are among world leaders, and quantum sensing (NV centers, magnetometry) where Russian-led teams publish cutting-edge results. These strengths can compensate for gaps in, say, semiconductor fabrication where Russia historically lags the West.
However, Russia also faces significant challenges relative to the top quantum powers:
Funding and Resources: While hundreds of millions of dollars have been committed to quantum tech, this is still an order of magnitude less than what the U.S. (which has billions through government agencies and companies) or China (reportedly over $10 billion in quantum investments) are spending. This means Russia must spend smartly and cannot afford large-scale failures. Access to the latest equipment is also costly; advanced microelectronics, cryogenic systems, and lasers often have to be imported.
Sanctions and Export Controls: Since 2014 (and especially after 2022), Russia has been under increasing export controls that limit its ability to import high-tech components. “International restrictions” have posed “significant challenges” to tech development, as Yunusov acknowledged. For quantum research, critical items include superconducting electronics, certain laser systems, dilution refrigerators, and networking gear – many of which are made by Western firms. Russia has managed by turning to alternative suppliers (for instance, some cryostats can be bought from Finland’s BlueFors, as indicated by references on RQC media ) or developing domestic substitutes. But this environment forces delays and can isolate Russian scientists from full participation in global supply chains and collaborations. On the positive side, it has pushed Russia to seek more self-reliance in quantum tech – spurring initiatives in indigenous material development and hardware manufacturing, as noted by the emphasis on “technological sovereignty” in materials.
Talent Drain vs. Retention: Russia boasts many skilled physicists and mathematicians, but historically many would work abroad for better opportunities. The quantum initiative has tried to reverse this through megagrants and inviting foreign experts, yet there is an ongoing risk that top young talent might migrate to labs in the US or Europe if they offer better funding or stability. Political developments have made international mobility more complicated recently, but also foreign scientists’ willingness to collaborate in Russia has decreased in some cases. Ensuring a pipeline of trained personnel who stay in-country (or return to country) is an ongoing challenge. The NQL’s outreach to involve foreign experts and train students domestically is one response.
Technological Gaps and Need for Validation: Some observers note that while Russia announces prototypes (e.g., a 50-qubit neutral atom computer), there is often limited public data on performance (error rates, algorithms run, etc.). This makes it hard to gauge how competitive these systems are against IBM or Google’s machines. The lack of peer-reviewed publications or open benchmarking for some of the Russian developments leaves a question mark. Achievements like the 50-qubit devices position Russia in the race, but “there is a need for transparency, peer review and public demonstrations to assess competitiveness,” as one analysis put it. In global science, credibility is built through such validation. Russia will need to increasingly engage in international conferences, publish results, and allow independent experts to test its claims to truly solidify its rank among the top quantum nations.
Relative to its competitors:
The United States leads in quantum computing hardware (IBM, Google, IonQ, Rigetti, etc.), software, and has significant programs in quantum networking and sensing (e.g., DOE quantum internet blueprint, DARPA projects). U.S. strengths are its massive private sector investment and its open academic culture which draws global talent. Russia cannot match the likes of IBM’s 433-qubit processors yet, but it is focusing on specific goals (50–100 qubits, certain use-cases). Notably, Russia has a more centralized approach whereas the U.S. is more distributed (academia, industry, multiple agencies). This centralization can sometimes accelerate projects (less redundancy), but it can also be less flexible.
China has made quantum tech a national priority with enormous funding, achieving breakthroughs like a 76-photon quantum supremacy experiment and a 2,000 km Beijing-Shanghai QKD backbone plus the Micius satellite. In some areas, China is ahead of everyone (quantum communication deployment). Russia has wisely partnered with China on satellite QKD, effectively piggybacking on Chinese infrastructure to perform joint feats. However, in quantum computing, China’s best labs (USTC, Alibaba, Baidu, etc.) have also demonstrated 60+ qubit superconducting chips and advanced photonic computers, which likely still exceed Russian capabilities in certain metrics. Russia’s approach of multiple smaller platforms might yield competitive results, but it lacks a titanic effort equivalent to China’s construction of entire quantum research megacenters. That said, culturally and politically Russia finds it easier to align with China now, which could mean more technology sharing and collaboration that benefits both in competing with the West.
The European Union and UK have strong quantum programs (EU’s €1 billion Quantum Flagship, Germany’s €2 billion program, etc., plus companies like IQM, Pasqal, Quantinuum in the UK). Europe excels in specific areas: ion traps (Austria, UK), photonics (France, Germany), and has established quantum startups. Pre-2022, Russia had substantial collaboration with European scientists (many RQC researchers had European ties, and joint projects existed under EU frameworks). Those have been curtailed recently, isolating Russia from some of the European ecosystem. This is a disadvantage because science thrives on collaboration. Still, Russia’s presence in international conferences (like hosting the annual ICQT quantum conference in Moscow up to 2019) built a foundation of contacts that may revive in the future. Relative to Europe, Russia’s strengths are perhaps more in quantum-resistant cryptography (Europe has focused less on PQC, more on QKD) and possibly in certain sensor domains, whereas Europe’s industrial base for building quantum hardware (e.g., Finland’s cryogenics, Dutch lithography) is stronger.
In summary, Russia is emerging as a significant “third player” in quantum tech, after U.S. and China, on par with or slightly behind the EU collective. It has proven capable of major advances – like the 50-qubit machines – “despite external challenges” like sanctions. Its strategy of self-reliance plus selective cooperation (with China and other friendly nations) is shaping a distinct path. Domestically, the quantum program has fostered a sense of competition and pride – Putin’s public interest in it trickles down as pressure for results, which can be a powerful motivator (witness the scientists racing to meet the 2024 deadline for 50 qubits). Yet, to truly claim quantum supremacy or leadership, Russia will need to demonstrate sustained innovation and perhaps a breakthrough that others haven’t achieved. It is worth noting that quantum technology is not a zero-sum game; Russia can lead in some areas while following in others. For example, it might not produce a 1000-qubit quantum computer before IBM, but it could lead in making a deployed quantum-secure communication network across a large region, or in integrating quantum sensors in military applications ahead of others.
Outlook
Looking ahead, Russia’s quantum technology initiatives are poised to enter a new phase. With the initial 2019–2024 roadmap period concluding, a follow-up program for 2025–2030 is likely to be launched (if not already in planning), building on the successes and lessons so far. We can expect several developments in the coming years:
Scaling Up Quantum Computers: The immediate technical goal is to realize a ~75-qubit universal quantum computer by 2025. Achieving this will involve enhancing qubit coherence and two-qubit gate fidelities toward the 99.9% mark, as well as implementing rudimentary quantum error correction. Russian teams will probably attempt a cloud-based quantum computing service once a stable NISQ (noisy intermediate-scale quantum) device is available – perhaps offering access to a 50-qubit machine for select research or industry partners. By 2030, Russia aims for quantum computers that “surpass classical supercomputers in specific calculations.” This suggests a focus on quantum advantage demonstrations in areas like quantum chemistry, optimization, or machine learning. To get there, research will intensify on quantum interconnects and modular architectures (linking multiple 50-qubit modules, for example), as well as on software – developing algorithms tailored to whatever qubit counts and error rates are achievable. We will likely see Russia invest more in quantum software startups or groups (to ensure useful applications are ready when the hardware matures) and in fabrication capabilities (maybe a dedicated fab line for superconducting qubits or silicon spin qubits to improve yield and design cycle).
Industrial and Economic Impact: As quantum hardware improves, Russia will push for its use in solving practical problems. Sectors like oil & gas (for materials and reservoir simulations), pharmaceuticals (for drug molecular modeling), logistics (optimization problems relevant to Russia’s vast supply chains), and finance (risk and portfolio calculations) are probable early adopters. We can expect pilot projects where a quantum algorithm is tested side-by-side with classical HPC to see if it offers an advantage – for example, Rosatom might use a quantum computer to model novel materials for reactors or batteries, given its interest in materials science for “technological sovereignty.” The government will also encourage the formation of quantum tech startups to commercialize spin-off technologies from the research labs. By 2030, Russia hopes not just to have built quantum machines, but to have a domestic quantum industry that contributes to the economy (much like the space and nuclear industries do).
Expansion of Quantum Communication Networks: On the communications front, the next years will likely see the quantum network infrastructure in Russia expand from pilot routes to a wider national quantum-secured network. The 7,000 km of QKD links planned by 2024 will probably extend further, possibly aiming to connect all major cities in the European part of Russia and some in Siberia. There is also likely to be a push to integrate quantum communication into the government’s secure communications channels (perhaps the diplomatic cable network or military communication lines) to future-proof them. Russia may launch its own quantum satellite to securely link to allies or cover its vast territory – reports have circulated about Roscosmos considering a quantum satellite project (especially after seeing China’s success). Internationally, Russia will continue to collaborate with China and potentially India and other BRICS nations on a quantum communications alliance. This could mean that by late 2020s, a quantum-encrypted channel exists between Moscow, Beijing, Delhi, etc., creating a sort of “quantum cryptography club.” Such developments might spur a parallel move in the West (e.g., a NATO quantum communication network), but Russia’s early involvement with China positions it well in this sphere. Also, by embracing post-quantum cryptography early, Russia could update its national encryption standards (GOST algorithms) to quantum-resistant versions well before large quantum computers appear. We might see, for instance, the Russian government mandate a switch to lattice-based encryption for all government data by a certain year, to mitigate the risk of storage and later decryption by foreign quantum computers. This proactive stance would be in line with its emphasis on securing “long-term data protection” in the quantum era.
Quantum Sensors Deployment and New Capabilities: Many quantum sensor projects in Russia are moving from research toward implementation. In the coming years, some of these will likely be productized or field-tested. For example, the compact optical clock for GLONASS could be launched on a test satellite to validate its performance in orbit. If successful, the next generation of GLONASS (mid/late 2020s) may feature onboard quantum clocks, giving Russia the most accurate navigation system in the world (a strategic and commercial boon). Quantum magnetometers and gravimeters might be tested in exploration – Rosneft or Gazprom could use quantum sensors to survey oil fields with greater sensitivity than classical instruments, potentially discovering resources more efficiently. The defense sector may trial quantum inertial navigation units in naval vessels or aircraft – initially as supplementary units alongside conventional gyros, but with the aim to eventually rely on them for independent navigation. By 2030, a Russian ballistic missile submarine might navigate for months without GPS by using a quantum accelerometer-based navigation system, for instance. On the scientific front, Russia’s contributions to global projects (like monitoring fundamental constants, improving time standards for astronomical observatories, etc.) will raise its profile. Quantum sensing is an area where breakthroughs often lead to Nobel Prizes; if Russia continues strong research (as with the NV center work), it could achieve landmark results that boost its scientific prestige.
Overcoming Challenges and Potential Course-Corrections: The outlook isn’t without uncertainties. Russia will need to mitigate the impact of ongoing sanctions – this might involve increasing cooperation with non-Western suppliers (China can provide some advanced technology, though China itself is careful with sharing critical tech). There’s also the question of maintaining international collaboration in a divided geopolitical climate. Science benefits from open collaboration, and one hopes that quantum research can remain a bridge (for example, Russian theorists still co-author papers with Western colleagues). A positive sign is that quantum tech has civilian benefits that encourage keeping channels open. If the political situation allows, we might see a normalization where Russian labs rejoin European projects (perhaps via neutral channels or under larger frameworks like BRICS science cooperation). If not, Russia will double down on self-reliance and building a domestic supply chain for quantum tech – meaning investments in indigenous cryogenics, nanofabrication, and photonics manufacturing capabilities. The emphasis on new materials indicates a long-term strategy to not depend on Western semiconductor tech.
In conclusion, Russia’s quantum technology initiatives have moved from planning to implementation with notable speed. In a span of about five years, Russia went from organizing its fragmented expertise to demonstrating quantum computers and quantum networks that place it in the top tier of nations in this field. The coming years will test its ability to scale and integrate these technologies into usable systems that benefit its economy and security. If Russia can maintain momentum – training new specialists, funding the costly experimentation, and fostering innovation despite international headwinds – it is poised to remain a key player in the unfolding “second quantum revolution.” Observers can expect Russia to announce more milestones (e.g., a 100-qubit device, or a country-wide quantum-secured communication network) between now and 2030. Its approach, characterized by central coordination, strategic focus on security, and a strong scientific tradition, offers an alternative model to the more commercially driven quantum development in the West.