Quantum Technology Initiatives in Europe and EU
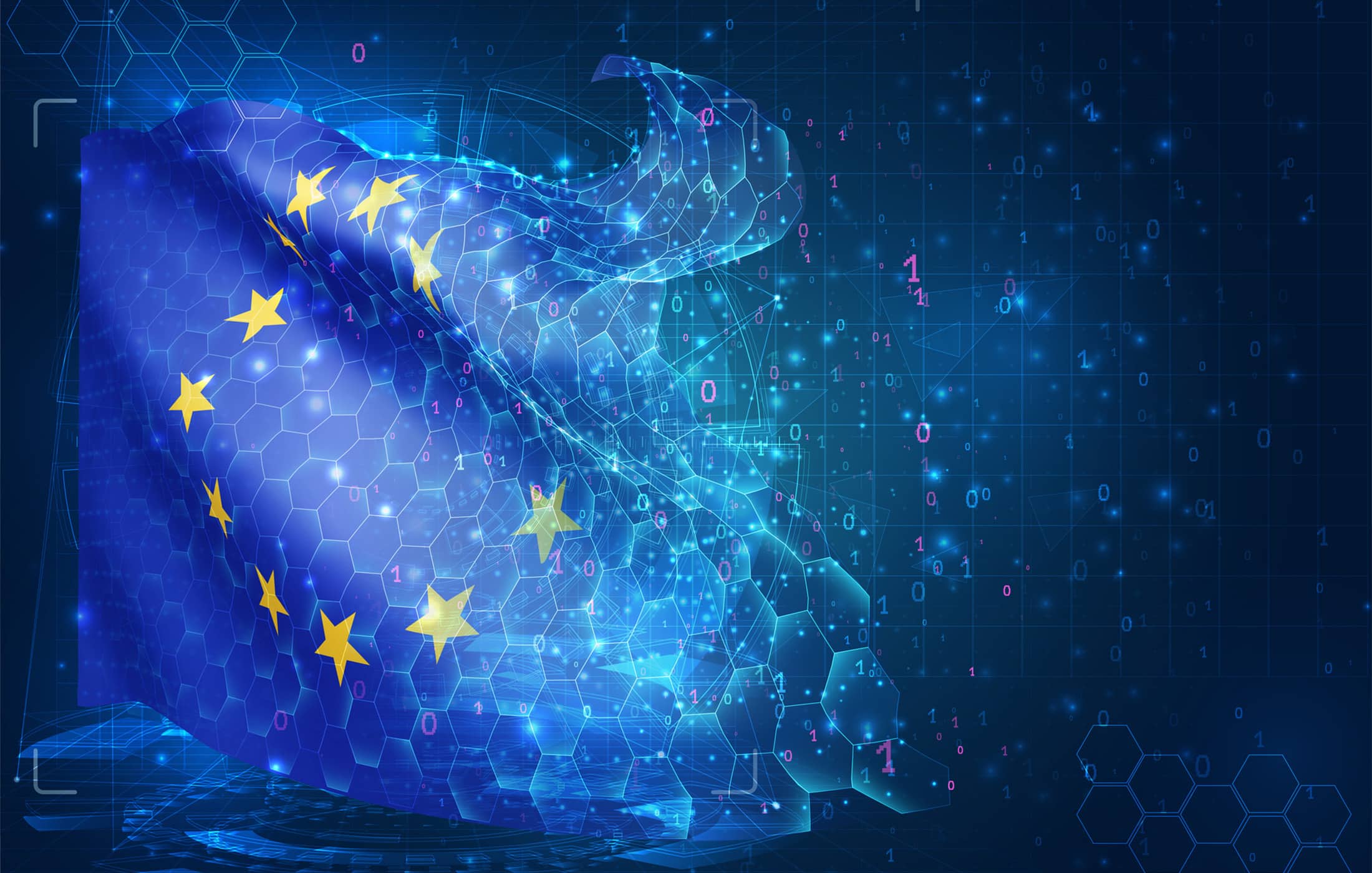
Table of Contents
Introduction
European involvement in quantum research dates back several decades, with EU-wide collaborations steadily growing since the early 2000s. Over the years, the European Commission funded a series of projects on quantum information and technology under its Framework Programs, laying groundwork for today’s efforts. A watershed moment came in 2016 with the Quantum Manifesto, a call to action endorsed by over 3,500 scientists and industry stakeholders across Europe. This manifesto galvanized political support and led to the launch of the EU Quantum Technologies Flagship in 2018 – a €1 billion, 10-year initiative aimed at keeping Europe at the forefront of the “second quantum revolution.” The Quantum Flagship followed in the footsteps of other EU large-scale science programs (like the Graphene Flagship and the Human Brain Project) and marked one of the EU’s most ambitious research investments to date.
Since its launch, the Quantum Flagship has funded a portfolio of R&D projects spanning quantum computing, communications, simulation, metrology, and enabling technologies. In the 2018–2021 ramp-up phase alone, it supported 20 major projects and engaged over 5,000 researchers across Europe. This coordinated approach sought to leverage Europe’s longstanding scientific excellence – indeed, over 50% of academic papers in quantum physics in the mid-2010s had European authors – and translate it into technological leadership. Early milestones included demonstrators like OpenSuperQ, a pan-European effort to build a 100-qubit superconducting quantum computer hosted at Jülich, Germany. By consolidating national strengths into a collective strategy, the EU aimed to kick-start a competitive European quantum industry and avoid lagging behind the U.S. and China.
Quantum Computing Initiatives and Advancements
Pan-European Programs: The Quantum Flagship and Beyond
At the heart of Europe’s quantum computing push is the EU Quantum Flagship. With a long-term vision of a “Quantum Web” – where quantum computers, simulators and sensors are networked via quantum communication – the Flagship supports research from basic science through to industrial prototypes. During its first phase, projects under the Flagship achieved notable successes. For example, the OpenSuperQ consortium (coordinated by Saarland University with partners like ETH Zurich and Forschungszentrum Jülich) developed a prototype superconducting quantum processor and associated infrastructure, positioning Jülich to host one of Europe’s first home-grown quantum computers. Other EU-backed efforts explored alternative technologies: AQTION advanced trapped-ion processors, while PASQuanS and its successor PASQuanS2 (led by the Max Planck Institute of Quantum Optics) built programmable quantum simulators using neutral atoms for solving physics and chemistry problems. By 2021, the Flagship had supported 19 projects covering the four pillars of quantum computing, simulation, communication, and sensing – with organizations like CNRS participating in many of them.
The EU is also integrating quantum computing into its broader digital infrastructure plans. Under the EuroHPC Joint Undertaking and projects like HPCQS, Europe is deploying hybrid high-performance computing (HPC) systems that couple classical supercomputers with quantum processors. In 2024, the French startup Pasqal delivered a 100+ qubit neutral-atom quantum processing unit (“Orion”) to France’s CEA-GENCI supercomputing center, with a twin system to be installed at the Jülich Supercomputing Centre in Germany. These machines will be connected to conventional supercomputers (Joliot-Curie in France and JURECA in Germany) to create cloud-accessible quantum-HPC testbeds for European users. By the end of 2024, this initiative will realize two operational quantum-enhanced supercomputing environments, exemplifying the EU’s strategy of leveraging quantum accelerators for practical applications in optimization, chemistry, and machine learning.
National Strategies: Germany, France, the Netherlands, and Others
In parallel to EU-wide programs, individual European countries have launched ambitious national quantum initiatives, often in synergy with the Flagship. Germany has made quantum technology a strategic priority, vowing to become a “world leader” in the field. The German government earmarked €2 billion for quantum research and industry as part of its 2020 economic stimulus package. By 2024, Germany had already invested these €2 billion in a variety of programs, from basic research to startups, and opened an IBM Quantum Computation Center in Ehningen in partnership with Fraunhofer. This center hosts state-of-the-art IBM quantum systems on European soil, giving German and EU researchers cloud access to cutting-edge hardware. Germany’s strategy includes funding indigenous quantum hardware too – for example, Infineon is jointly developing ion-trap processors with the startup eleQtron – and fostering regional hubs in Munich, Ulm, and other cities for quantum science and engineering.
France likewise launched a comprehensive Quantum Initiative in January 2021. Backed by €1.8 billion over 2021–2025, the French quantum plan spans quantum computing, communications, cryptography, and sensing. It is funded through the France Relance recovery plan and the Programme d’Investissements d’Avenir (PIA4), reflecting high-level political commitment even amid economic challenges. France’s strategy builds on its strong research legacy – from Nobel-winning physicists like Serge Haroche and Alain Aspect to applied efforts by companies like Atos. The plan devotes substantial support to developing quantum computers and simulators, with aims to coordinate academia (CNRS, CEA, INRIA) and industry, and to train a new generation of “quantum engineers.” Notably, Atos has developed the Atos Quantum Learning Machine, a high-performance quantum simulator, and is involved in collaborative efforts to build a French quantum computer. By structuring a national ecosystem and investing in enabling technologies (e.g. cryogenics, semiconductors), France seeks to bolster its sovereignty in quantum tech as U.S. and Chinese firms race ahead.
The Netherlands has positioned itself as a quantum hotspot through the Quantum Delta NL program. In April 2021, Quantum Delta NL was awarded €615 million from the Dutch National Growth Fund to strengthen the country’s quantum ecosystem. This investment supports a multi-year agenda focused on R&D, talent development, and startup growth, with the explicit goal of making the Netherlands a “Silicon Valley” of quantum in Europe. The Dutch quantum hub, centered around Delft, Leiden, Amsterdam and Eindhoven, boasts world-class groups in quantum computing and communication. TU Delft’s QuTech, for instance, leads research on quantum internet protocols and demonstrated one of the first multi-node quantum networks. The Netherlands also launched Quantum Inspire, Europe’s first public quantum computing platform in the cloud, allowing users to run algorithms on a small superconducting and silicon-spin qubit processors. Dutch researchers remain at the forefront of quantum error correction and hardware (for example, pioneering work on semiconductor-based qubits and quantum repeaters), leveraging the country’s tight-knit network of universities and companies.
Other EU nations have their own programs contributing to the continental effort. Finland, for example, invested in a partnership between state research center VTT and Finnish startup IQM to build the nation’s first quantum computer. By 2022 they achieved a 5-qubit device, scaled to 20 qubits in 2023, and in 2025 a 50-qubit superconducting quantum computer – one of the first in Europe at that scale – was made operational in Espoo. This project, supported by over €20 million in public funding, underlines how smaller countries are also making significant contributions. Austria, Italy, Spain, and Scandinavia likewise invest in quantum research (often focusing on niches like photonic quantum computing or quantum software) and participate in EU-wide collaborations. Switzerland, while not in the EU, is deeply integrated in Europe’s quantum landscape – ETH Zurich and EPFL are top institutes, and Swiss companies (e.g. ID Quantique) have been early leaders in quantum cryptography.
Leading Research Institutions and Academic-Industry Collaboration
Europe’s quantum advancements are underpinned by a dense network of top-tier research institutions. The Max Planck Society in Germany, for instance, hosts multiple institutes driving quantum science – from the Max Planck Institute of Quantum Optics (MPQ) which has produced record-breaking photon entanglement sources, to the MPI for Solid State Research exploring quantum materials. The French CNRS coordinates dozens of labs working on quantum theory, optics, and nanotech, providing a vast pool of expertise and a structure for multi-institution projects. Universities such as ETH Zurich and TU Delft rank among the world’s elite in quantum computing research, contributing hardware breakthroughs (ETH has world-leading groups in superconducting circuits and quantum architecture) and novel algorithms. These institutions frequently collaborate across borders: for example, ETH Zurich and Forschungszentrum Jülich work together in the OpenSuperQ project to design Europe’s superconducting processors. Such cooperation is amplified by EU networks like the Quantum Community Network (QCN) set up to link stakeholders across all member states.
Crucially, Europe has been fostering academia–industry links to translate research into innovation. One approach is hosting foreign quantum systems as a springboard: the partnership with IBM that brought an IBM Quantum System One to Fraunhofer in Germany gave companies and researchers hands-on experience with a 27-qubit machine in 2021, accelerating skills development. Conversely, European industry is now producing competitive hardware of its own. Startups have proliferated out of research labs, often supported by Flagship grants or national incubators. Notable examples include Pasqal (France), founded by Institut d’Optique researchers, which builds neutral-atom quantum processors now scaling beyond 100 qubits. IQM (Finland/Germany), spun out of Aalto University, focuses on superconducting quantum computers and has delivered systems for both research and cloud services. Alice&Bob in Paris is developing “cat qubits” for fault-tolerant superconducting machines, while Quandela (France) is building photonic quantum computing modules. Large European technology firms are also active: Atos provides quantum simulation tools and partners with national labs on computing and algorithms; Siemens, Airbus, and Bosch are exploring quantum computing use cases in optimization and materials; Thales and Infineon are researching enabling technologies like quantum-safe encryption chips and control electronics. This growing private-sector involvement complements Europe’s academic strength, although scaling startups into “quantum unicorns” remains an acknowledged challenge in the EU’s ecosystem.
Quantum Communications and Cryptography in Europe
Building a Continental Quantum Network: QKD and the Quantum Internet
Secure quantum communication is a pillar of Europe’s quantum strategy, where the EU already has considerable expertise. European researchers performed some of the earliest demonstrations of quantum key distribution (QKD) in fiber and free-space, and several EU companies (ID Quantique in Switzerland, Aurea Technology in France, Toshiba’s Cambridge lab, etc.) have commercial QKD systems. To move from experiments to infrastructure, the EU launched the European Quantum Communication Infrastructure (EuroQCI) initiative in 2019. By 2022, all 27 EU member states had signed on to EuroQCI, committing to deploy an ultra-secure quantum network spanning the Union. This network will integrate terrestrial fiber-optic quantum networks with satellite QKD links, adding a quantum layer of security to Europe’s communications. The vision is to connect government institutions, critical infrastructure, and eventually businesses and individuals with quantum-encrypted links immune to eavesdropping. Initial steps are already funded under the Digital Europe programme: national QKD testbeds are being rolled out, and cross-border fiber links are planned to start interconnecting these networks in the next couple of years. The European Commission aims for the EuroQCI to be fully operational by 2027, roughly aligning with anticipated advances in quantum computing that could threaten classical cryptography.
A flagship endeavor within EuroQCI is the development of quantum communication satellites. The European Space Agency, in partnership with the EU, is overseeing EAGLE-1, which will be Europe’s first quantum key distribution satellite. EAGLE-1, led by an industrial consortium (SES and others), is slated for launch around 2025–2026. It will test distribution of encryption keys from low-Earth orbit to ground stations across Europe, validating space-based QKD technology for the EuroQCI system. This follows on China’s pioneering Micius quantum satellite; Europe’s entry will ensure the EU keeps pace in space-based secure communications. In the interim, European scientists have not been idle – they set world records for long-distance entanglement distribution and quantum teleportation through fiber, and tested quantum equipment in stratospheric balloon flights. Ground networks are progressing as well: SECOQC, a Vienna-led project, built one of the first metropolitan QKD networks years ago, and more recently a Quantum Internet Alliance (another EU Flagship project) is working on enabling quantum entanglement swapping between network nodes to eventually realize a genuine quantum internet. In 2022, researchers at Delft (QuTech) demonstrated a three-node network with entangled qubits shared between distant nodes, an important step toward larger quantum networks. These efforts underscore Europe’s strength in quantum communications research, although practical deployment at scale will depend on continued engineering and investment via EuroQCI.
Advances in Quantum Cryptography and Post-Quantum Security
Europe is not only using quantum physics for secure communication, but also preparing for a future where quantum computers threaten classical cryptosystems. Quantum-resistant cryptography (post-quantum cryptography, PQC) has been a focus of European researchers for many years. Notably, European teams played a key role in developing several of the algorithms recently selected by NIST as global PQC standards. For example, the CRYSTALS-Kyber encryption algorithm and CRYSTALS-Dilithium digital signature scheme – two of NIST’s chosen post-quantum algorithms – were co-created by researchers from Radboud University (Netherlands) and partners at CWI Amsterdam, ENS Lyon, IBM Zurich and others. Similarly, the general lattice-based approach to cryptography has deep roots in European mathematics and computer science. This transatlantic collaboration paid off when NIST announced in 2022 that Kyber and Dilithium (along with another scheme SPHINCS+) will form the core of next-generation cryptographic standards. The European involvement in these algorithms ensures the EU has in-house expertise on the tools likely to secure global communications in the coming decades.
On the policy side, the EU has been proactive about quantum-proof security. The European Telecommunications Standards Institute (ETSI), headquartered in France, was one of the first bodies to form a working group on quantum-safe cryptography, and it continues to develop implementation standards for PQC and QKD globally. The EU’s cybersecurity agency ENISA has issued recommendations urging industries and governments to begin planning for a transition to PQC, following the principle of “crypto agility” to swap out vulnerable algorithms before quantum computers arrive. Several Horizon 2020 research projects (like PQCRYPTO and FutureTPM) and current Horizon Europe projects focus on cryptographic techniques that can resist quantum attacks, as well as practical challenges like integrating new algorithms into secure elements and public-key infrastructures. Furthermore, European companies (e.g. Infineon, Siemens) are exploring post-quantum security for critical systems such as smart cards, automobiles, and industrial control, often in collaboration with academic cryptographers. Europe’s emphasis on data privacy and regulations (like GDPR) also drives its strong interest in quantum-safe encryption – ensuring that sensitive European data remains secure against adversaries armed with quantum computers in the future. In summary, Europe’s strategy on quantum cryptography is two-pronged: deploy QKD where high security is needed in the near term, and simultaneously upgrade classical crypto infrastructure with post-quantum algorithms for long-term, ubiquitous security.
Quantum Sensing and Metrology Developments
Quantum sensing is a third major pillar of Europe’s quantum technology drive, promising breakthrough improvements in measurement, imaging, and navigation. Quantum sensors exploit phenomena like superposition and entanglement to measure physical quantities (time, acceleration, magnetic fields, etc.) with unprecedented precision, beyond what classical sensors can achieve. European research institutions and companies have been at the forefront of this field, often translating fundamental physics advances into real-world instruments.
One area of focus is inertial sensing for navigation – using quantum interferometry to build ultra-precise accelerometers and gyroscopes. In 2022, the French company Exail (formerly iXblue) demonstrated the world’s first 3-axis quantum accelerometer for navigation, developed with the LP2N laboratory in Bordeaux. This device uses cold atom interferometers along three perpendicular axes to measure acceleration without drift, achieving a continuous output with 50 times better accuracy than standard high-end inertial systems. The achievement, published in Science Advances, is a step toward drift-free inertial navigation units that could allow submarines, aircraft, or autonomous vehicles to navigate precisely for long periods without GPS. European defense and aerospace organizations are keenly interested – for instance, France’s and Germany’s defense research programs invest in quantum inertial sensor prototypes to maintain strategic autonomy in navigation. The EU’s stability and security could benefit from such sensors in everything from civilian airliners to space probes.
In fact, quantum sensing is extending to space applications through projects like CARIOQA (Cold Atom Rubidium Oscillator in Orbit for Quantum Accelerometry). Kicked off in 2024 with support from the European Commission, CARIOQA will develop a space-qualified cold-atom accelerometer and aims to test the first quantum accelerometer in orbit by 2030. Led by a consortium including France’s CNES, Germany’s DLR, and Airbus Defense & Space, this mission will demonstrate ultra-sensitive measurements of Earth’s gravity field from space. The ability to detect minute gravity variations has huge implications: it can improve the tracking of water resources, map subterranean structures, and enhance our understanding of geophysical processes. Future satellite constellations with quantum gravimeters could monitor climate change effects (ice loss, sea level, aquifer depletion) with unprecedented resolution. The CARIOQA project exemplifies how Europe is leveraging its excellence in atomic clocks and cold atom physics – traditionally strong fields in Paris, London (excluded here), Berlin – to create next-generation Earth observation tools. ESA is also planning to fly a cold atom clock experiment on the ISS and has included quantum sensors in its technology roadmap.
Back on the ground, European labs have built some of the world’s best atomic clocks and frequency standards, which are quantum devices underpinning precision timekeeping and navigation (e.g., for Galileo satellites). Institutions like PTB (Germany) and SYRTE (France) lead in optical lattice clocks that are so precise they lose less than a second over the age of the universe. Such clocks are not only vital for timekeeping; when connected via fiber links, they can measure differences in gravitational potential between cities (relativistic geodesy), effectively using time as a probe for height and mass distribution. The Quantum Flagship’s metrology projects and the European Metrology Programme are supporting the development of transportable optical clocks and quantum gravimeters. In the UK (excluded from this scope) and Italy, portable quantum gravity sensors have already detected underground tunnels by sensing tiny gravitational changes. Similar devices are under development in France and Germany, aiming at civil engineering and defense applications like detecting hidden structures or ensuring the integrity of critical infrastructure.
Another impactful area is quantum magnetic sensors and imaging. Using NV-centers in diamond (a solid-state qubit) or cold atomic vapors, European researchers have created magnetometers sensitive enough to detect brain neuron signals (MEG) and heart electrophysiology without contact. The Flagship project ASTERIQS, for example, focused on diamond-based quantum sensors, aiming at applications ranging from biomedical imaging to material analysis. European startups like Quside (Spain) and Magnify (Germany) are productizing such sensors for use in healthcare and automotive industries. In scientific research, quantum optics techniques are enhancing detectors used in experiments like gravitational-wave observatories (using squeezed light to improve sensitivity of LIGO/Virgo, an area where GEO600 in Germany made contributions). The breadth of quantum sensing work – from fundamental physics tests of the equivalence principle to everyday uses like navigation – highlights a strength of Europe’s approach: leveraging high-precision engineering and physics talent for both cutting-edge science and practical solutions.
Europe’s Global Position in Quantum Technologies: Strengths and Challenges
Europe enters the quantum race with distinct advantages as well as obstacles when compared to its global competitors. On the strengths side, the EU and associated European countries have a deep talent pool and a rich scientific heritage in quantum physics. European researchers have won multiple Nobel Prizes in quantum science (recently Alain Aspect in 2022 for entanglement experiments), and European institutions produce a large share of the world’s top quantum research – over half of the highly cited quantum science papers in the mid-2010s came out of Europe. This intellectual leadership is supported by robust public funding: the EU’s €1 billion Quantum Flagship and various national programs (Germany’s €2 billion, France’s €1.8 billion, etc.) mean Europe is among the world leaders in government investment in quantum tech. By setting clear strategic agendas and funding mechanisms, European governments have signaled that achieving “quantum sovereignty” is critical for economic and security reasons. Additionally, Europe excels in collaborative frameworks – the ability to bring together universities, research institutes, and companies across different countries is a comparative strength. EU-funded networks and projects enable sharing of expertise and resources continent-wide, something more fragmented efforts elsewhere may lack.
However, Europe also faces significant challenges in turning its quantum potential into sustained leadership. A 2022 analysis by Boston Consulting Group starkly noted that while the EU leads in public plans and talent, it lags the U.S. (and even China) in private-sector drive. The United States currently leads on most metrics, especially in commercial activity – American tech giants and start-ups have achieved larger quantum computers, accumulated more patents, and attracted far greater venture capital than their European counterparts. China, with massive state-backed programs, is not far behind the U.S. and even surpasses others in certain areas like quantum communication deployment. Europe’s fragmentation is often cited: despite the EU-wide Flagship, many national efforts still operate in silos and lack coordination, leading to duplication or inefficient use of resources across countries. This stands in contrast to the more unified national strategies seen in the U.S. (with federal coordination plus giants like IBM/Google) and in China (with centralized megaprojects).
Moreover, Europe’s innovation ecosystem for deep-tech startups is traditionally weaker in scaling up companies. The venture capital gap means European quantum start-ups often struggle to find late-stage funding and may relocate or seek investors abroad. There are few European companies with the might of Google, IBM, or Alibaba to pour billions into quantum R&D, so scaling largely relies on government support or midsize enterprises. The “brain drain” is a related concern: while Europe trains excellent quantum PhDs, many have been recruited by U.S. firms or choose to found companies in the U.S. where capital is abundant. BCG estimated that the U.S. has 2–3 times more quantum technology workers in industry than the EU, despite comparable numbers of research graduates. Ensuring that Europe’s talent stays and flourishes in local industries is an ongoing challenge.
In specific quantum domains, Europe’s global standing varies. In quantum computing hardware, Europe is competitive but not leading – U.S. companies have demonstrated devices with dozens to over a hundred high-quality qubits (and are racing toward error-corrected qubit networks), whereas European-led machines (IQM’s 54-qubit in Finland, Pasqal’s 100-atom system, etc.) are just reaching that scale now. Superconducting qubit leadership lies with U.S. and Chinese groups for now, though Europe’s focused efforts (like OpenSuperQ and national labs) may narrow the gap in coming years. In quantum communications, Europe has strong research but China took an early lead in deployment by launching the first quantum satellite and building extensive QKD networks between cities. EuroQCI and Eagle-1 will be Europe’s answer, likely making it a fast follower in QKD infrastructure by the mid-2020s. Notably, Europe’s emphasis on standardization and interoperability (through ETSI, EU framework programs) could become a strength if its QKD networks and PQC standards gain global adoption. In quantum sensing, the playing field is more level – Europe and China both excel (indeed, one assessment suggests China matches the U.S. in sensing, and Europe has comparable expertise). Europe’s work on atomic clocks, quantum navigation, and metrology is world-class, often in synergy with its thriving precision instrumentation industry.
Overall, the EU’s approach – a cohesive, long-term public investment coupled with academic excellence – gives it a solid foundation. Europe’s diverse strengths across all sub-fields (computing, communications, sensing) provide resilience; for instance, if superconducting qubits end up dominated by U.S. firms, Europe might lead in neutral atoms or photonic quantum computers as alternative routes. The challenges lie in speed and scale: the global race is intense, and Europe must translate research into real systems and markets faster. Initiatives to improve coordination (like the Quantum Flagship’s Coordination Office and the Quantum Community Network) and to inject more funding into startups (e.g. via the European Innovation Council or new public-private partnerships) are underway to address these gaps. The coming few years – often dubbed Europe’s “quantum decade” – will be critical in determining whether the EU can hold its own against the U.S. and China in this transformative technology domain.
Conclusion and Outlook
Europe’s quantum technology landscape has evolved from disparate academic projects into a coordinated multi-billion euro endeavor encompassing the EU and its member states. The historical commitment to quantum science is now manifesting in tangible outputs: prototype quantum computers in laboratories and supercomputing centers, quantum-secure communication testbeds linking cities, and quantum sensors poised to revolutionize measurements from under the Earth to outer space. The European Union’s flagship program and national quantum strategies in Germany, France, the Netherlands, and elsewhere have created a momentum that engages both prestigious research institutions (ETH Zurich, CNRS, Max Planck Society, etc.) and a growing quantum startup sector (Pasqal, IQM, Atos, and many more). This public-private ecosystem is steadily advancing the technical frontier in quantum computing – aiming for higher qubit counts and better error rates – while also translating quantum theory into real-world applications in communications security and precision sensing.
Looking ahead, the next 3–5 years will likely bring several milestone achievements for the EU’s quantum initiatives. By 2025–2026, Europe’s first quantum communication satellite Eagle-1 should be in orbit, joining terrestrial fiber networks to form an initial EuroQCI backbone and cementing Europe’s presence in secure quantum communications from space. Quantum key distribution services may start protecting sensitive government and financial data across a pan-European quantum network. In quantum computing, European-built processors are expected to grow in power: projects like OpenSuperQ+ are targeting 100+ qubit superconducting systems, French and German consortia are working on next-generation ion trap and neutral atom machines, and companies like IQM plan to deliver a 100+ qubit superconducting system by the mid-2020s. We can also anticipate the integration of quantum computers into national HPC centers to expand, following the Pasqal and IQM examples, giving researchers on-demand access to European quantum hardware via the cloud. This could spur algorithm development in optimization, cryptography analysis, drug discovery, and other fields that leverage quantum speed-ups.
In quantum sensing and metrology, Europe’s focus on practical devices means we might see the first field deployments of quantum gravimeters (for surveying or civil engineering) and portable atomic clocks used outside the lab. The CARIOQA project will progress, moving closer to a demonstration of quantum navigation in space by 2030, and ESA’s quantum technology program may produce novel sensors for planetary missions or climate monitoring. Meanwhile, ongoing R&D in magnetometry and imaging could yield spin-off medical technology (for example, quantum MRI enhancements) within European healthcare. These developments will benefit from Europe’s interdisciplinary collaboration – combining physics, engineering, and industry – which remains one of its core strengths.
Europe’s quantum future is not without uncertainty. Success will depend on sustaining funding and political support, nurturing talent and entrepreneurship, and tightening the collaboration among EU countries to avoid fragmentation. There are signs of progress on these fronts: the EU is contemplating a second quantum flagship or equivalent large program under the next funding framework, and new public-private partnerships (like national quantum innovation hubs) are being established to help startups scale. The competition is fierce, as the U.S., China, and others are also ramping up efforts. But the EU’s comprehensive approach – investing in quantum computing, communications, cryptography, and sensing in parallel – positions it well to exploit the synergies between these areas (for example, using quantum computers to design better quantum materials for sensors, or quantum networks to connect distributed quantum processors). European policymakers and scientists view the 2020s as a make-or-break period: by the end of this decade, quantum technologies should transition from lab curiosities to an enabling backbone of the digital economy. If current initiatives bear fruit, the EU and continental Europe will enter the 2030s with a secure quantum communication network, a nascent industry of quantum computing firms offering services, and quantum sensors enhancing everything from navigation to environmental science – firmly establishing Europe as a leading player in the quantum era.