Quantum Sensing – Key Use Cases
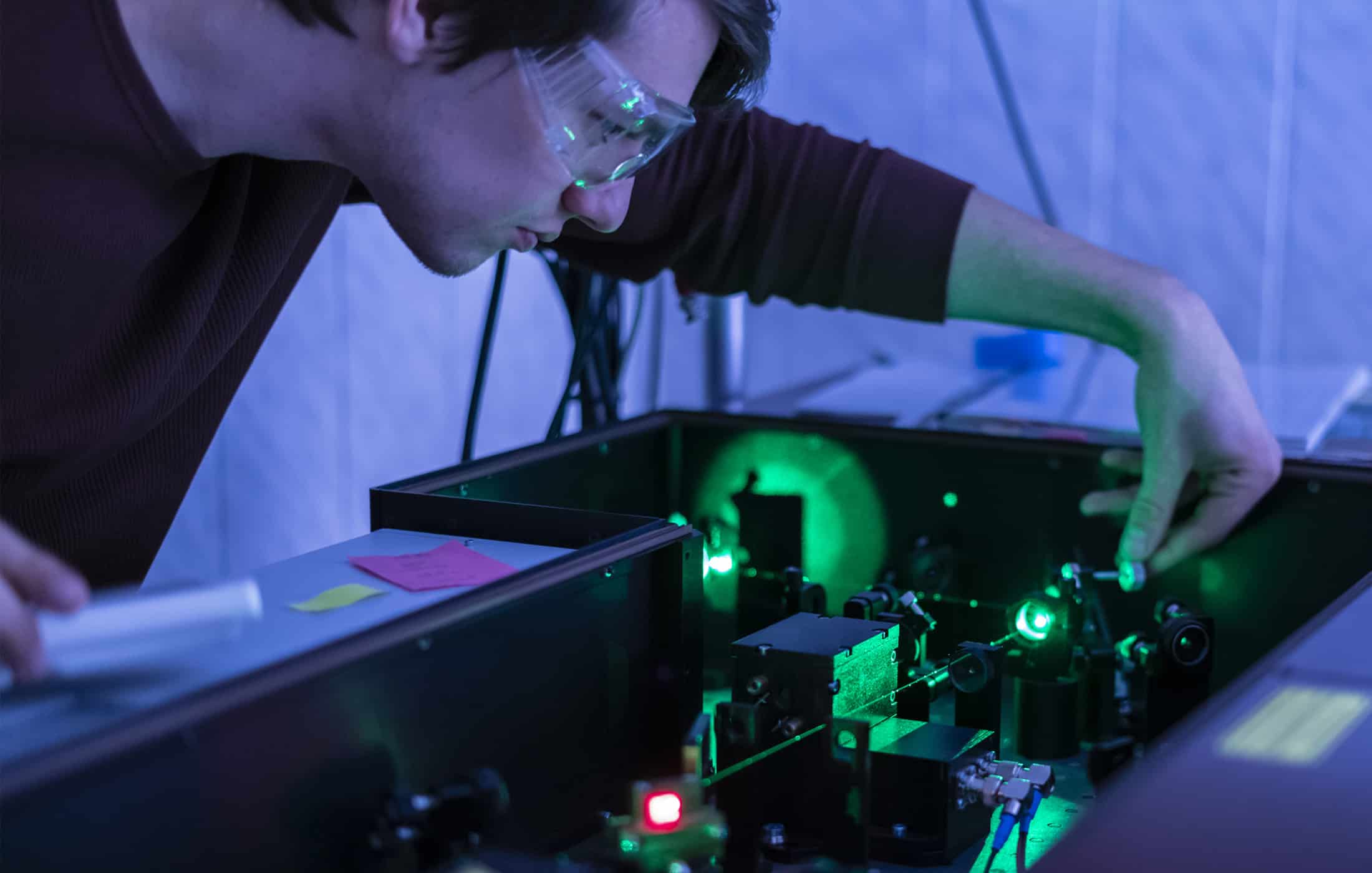
Table of Contents
Introduction
Imagine being able to see the invisible – detecting the faint magnetic whisper of a human brain in action, or sensing a submarine’s subtle disturbance of Earth’s gravity from miles away. Welcome to the world of quantum sensing, a breakthrough technology poised to redefine how we perceive reality. In this new era, sensors harness weird and wondrous quantum effects to achieve seemingly impossible precision. They can measure phenomena so faint or distant that classical instruments fall silent, much as a night vision scope reveals a starry sky invisible to the naked eye. Quantum sensing isn’t just an incremental improvement; it’s transformational – a leap that promises to unlock realms of detection once confined to science fiction.
At its core, quantum sensing goes beyond classical measurement limits. Traditional sensors – from thermometers to microphones – are ultimately constrained by thermal noise, electronic noise, and even the fundamental “shot noise” of particles. Quantum sensors break past these limits by exploiting the quirky properties of quantum mechanics, like superposition and entanglement. In a quantum sensor, particles (atoms, electrons, photons) are prepared in delicate quantum states that respond to minuscule changes in the environment. Because of this, quantum devices can detect tiny signals with precision beyond any classical strategy. For example, laser interferometers augmented with quantum‐entangled light have measured the ripples of spacetime from colliding black holes over a billion light years away. This level of sensitivity – literally nudging up against the limits set by Heisenberg’s uncertainty principle – is what makes quantum sensing so profound. It’s as if nature gave us a noise floor, and now we’ve found a way to push below it, revealing a whole new layer of subtle phenomena.
This paradigm shift didn’t happen overnight. The evolution of sensing technology spans millennia – a rich history of human ingenuity in extending our senses. In ancient China, the invention of the compass (a magnetized needle on water) was humanity’s first device to perceive Earth’s invisible magnetic field. The telescope and microscope in the 17th century expanded our vision to the vastness of Jupiter’s moons and the intricacy of microscopic life. Fast forward to the 20th century: quantum physics quietly entered everyday sensors. The first “quantum revolution” in technology gave us the transistor, the laser, and the MRI scanner, all reliant on quantum principles. These inventions were landmark breakthroughs – suddenly we could image the inside of the human body or build computers, feats unimaginable to earlier generations. Yet, devices like MRI and digital cameras, while quantum-enabled, still operate within essentially classical limits of measurement. Today’s quantum sensors mark a second revolution: for the first time, sensors are explicitly designed to leverage fragile quantum effects themselves. By using entangled photons, ultra-cold atoms, and other quantum resources, we are pushing into a new frontier of precision and miniaturization. In short, we’ve gone from sensing with ever better tools of classical physics to sensing with quantum physics – a profound shift in approach. The stage is set for quantum sensing to transform industries and scientific discovery in the coming decades, much as electricity or digital technology did in the past.
Transformative Use Cases Across Industries
Quantum sensing’s transformative potential is not confined to labs – it spans a broad range of industries, promising dramatic improvements in how we diagnose illnesses, secure nations, study our planet, and explore the cosmos. Below, we highlight some of the most impactful use cases of quantum sensors across key domains:
Healthcare: Imaging and Diagnostics Reimagined
Quantum Brain Imaging (Quantum MEG) – New quantum magnetometers are enabling magnetoencephalography (MEG) systems that can map brain activity with unprecedented sensitivity. Unlike conventional MEG that requires bulky cryogenic sensors, optically pumped quantum sensors can operate at room temperature, even in a wearable helmet. Researchers at the University of Nottingham have demonstrated a quantum-enabled MEG scanner that could herald a “new dawn” in the study of human brain function, detecting the faint magnetic fields of neural firing with greater mobility and comfort for patients. This could revolutionize how we diagnose and treat neurological conditions, from epilepsy to dementia, by capturing brain function in real time and high resolution.
Quantum-Enhanced MRI & Molecular Imaging – Quantum sensors are pushing medical imaging to molecular and even atomic scales. In Australia, scientists turned qubits (quantum bits) into miniature MRI sensors capable of imaging a single molecule’s atomic structure. This “quantum MRI” concept uses the spins of quantum probes (like nitrogen-vacancy centers in diamond) to detect magnetic signals from individual atoms in a biomolecule. The result is a 3D image of the molecule – something traditional MRI or X-ray crystallography cannot achieve. Such technology could allow us to peer into the fundamental building blocks of life, aiding drug discovery by revealing protein structures that were previously unobservable. As one researcher noted, a quantum MRI microscope for single molecules “could revolutionize how we view biological processes at the molecular level,” enabling early diagnostics and personalized medicine at the nanoscale.
Disease Diagnosis and Biotechnology – Beyond imaging, quantum sensors promise ultra-early detection of diseases through exquisite sensitivity. For instance, quantum biosensors might detect trace biomarkers or subtle changes in cells long before symptoms appear. In one example, diamond-based quantum magnetometers have been developed to pick up the tiny magnetic fields of heart and brain activity, opening the door to wearable medical monitors for heart arrhythmias or brain waves. Nanoscale spectroscopes leveraging quantum effects can identify chemical signatures of disease with extreme accuracy. In short, quantum sensing in healthcare could shift diagnostics from the macro (seeing tumors via MRI) to the micro, even molecular level – catching disease in its earliest, most treatable stages.
Defense & Security: A Quantum Leap in Sensing Capabilities
Quantum Radar for Stealth Detection – Stealth aircraft and encrypted communications may meet their match in quantum radar. Using entangled photon pairs, a quantum radar system can detect objects with much lower power and potentially defeat traditional radar jamming or stealth coatings. In a prototype demonstrated by researchers in 2020, entangled microwaves were used to detect a target, showing that quantum radars could eventually outperform conventional radar in certain scenarios. The entanglement allows the radar to sense a faint reflection by comparing it against its twin photon kept at the receiver – yielding a clear signal even amid noise. Such technology could enable detection of stealth bombers or hidden weapons with sensitivity far beyond today’s surveillance systems.
GPS-Independent Navigation (Quantum Compass) – Navigation systems are getting a quantum upgrade through ultra-precise inertial sensors. Quantum accelerometers and gyroscopes based on cold atoms can track position without any external GPS signal. In 2018, a UK team demonstrated a portable “quantum compass” – a self-contained quantum accelerometer that measures motion so precisely it can dead-reckon location over long periods. This device uses atoms cooled to near absolute zero and put into a quantum wave state (an atom interferometer) to measure acceleration with extreme accuracy. The payoff is navigation that cannot be jammed or spoofed – a critical advantage for submarines, aircraft, or even smartphones in GPS-denied environments. Military applications are obvious: vehicles could navigate covertly using an onboard quantum sensor, ensuring reliability even if satellites are knocked out.
Submarine & Threat Detection – The ocean’s depths hide nuclear submarines and other threats that are difficult to detect with conventional means. Quantum sensors offer new ways to sense disturbances in fields like gravity or magnetism to spot hidden objects. For example, quantum gravimeters can pick up minute fluctuations in Earth’s gravitational field caused by a large metal sub or underground bunker. Similarly, sensitive quantum magnetometers (using atomic vapor cells or superconducting circuits) can detect the faint magnetic signature of a vessel. Notably, quantum thermometers and barometers using cold atom techniques have been proposed to enhance sonar, enabling detection of submarines via subtle pressure or temperature anomalies. These approaches, combined with AI analysis, could dramatically improve situational awareness for defense. Even communication security stands to benefit: quantum sensing and communication blur together in quantum cryptography, where any eavesdropper’s attempt to intercept signals is itself detected by quantum means. Nations are investing in quantum sensing for espionage and counter-espionage – from sniffing out hidden nuclear materials to creating unhackable quantum communication links. In the security realm, quantum sensing is poised to enhance both the “sword and the shield,” providing more penetrating surveillance and more secure detection of illicit activities.
Space & Climate Science: Eyes on Earth and Beyond
Quantum Gravimeters for Earth Monitoring – Understanding climate change and managing resources require incredibly precise measurements of Earth’s gravitational and magnetic fields. Quantum sensing is stepping up to this challenge. Teams in Europe are developing space-based quantum gravimeters that use atom interferometers to track subtle changes in Earth’s gravity. These devices measure acceleration so precisely that from orbit, they can detect minute shifts in gravity caused by melting glaciers, shifting ocean currents, or depleting aquifers. In essence, a satellite equipped with a quantum accelerometer can map changes in mass distribution on Earth in real time. This provides a sharper view of climate change indicators – for example, tracking ice loss in polar regions or drops in groundwater levels with unprecedented precision. Such data is critical for climate science and policy, enabling early warnings for sea-level rise or drought.
Planetary Exploration & Space Navigation – Quantum sensors are also set to transform how we explore other planets and navigate in space. On future missions to the Moon or Mars, quantum gravimeters could help find subsurface water or lava tubes by spotting tiny gravity anomalies as a rover traverses the surface. Quantum magnetometers might map planetary magnetic fields in detail, shedding light on a planet’s interior and geology. For spacecraft navigation, quantum star trackers and inertial sensors could guide vehicles far from Earth without relying on Earth-based GPS. Even detecting gravitational waves in space – ripples from distant black hole mergers – might be achieved with networks of quantum clocks or atom interferometers onboard satellites. In short, quantum sensing extends to the final frontier: from monitoring Earth’s health from space to uncovering hidden features of other worlds, giving scientists new tools to study the dynamics of oceans, atmospheres, and planetary cores.
Environmental Monitoring – Back on Earth, quantum sensors will enhance how we observe our environment. Quantum lidar systems (light detection and ranging) use quantum light sources to create ultra-detailed 3D maps of terrain and forests, useful for tracking changes in ecosystems or ice sheets. Because of their improved resolution and lower noise, they can detect subtle shifts – like the slight bulge of landmasses due to groundwater changes. Quantum-enabled gas sensors could detect greenhouse gas concentrations at ppb (parts-per-billion) levels, improving climate modeling. These advances mean we can keep a closer watch on Earth’s vital signs. Just as weather satellites revolutionized meteorology, quantum sensor networks may revolutionize climatology, giving earlier alerts on everything from volcanic activity (via gravity changes) to approaching hurricanes (via pressure and magnetic field shifts).
Energy & Industry: Precision and Efficiency Boosts
Oil, Gas and Mineral Exploration – The energy industry stands to gain powerful new exploration tools through quantum sensing. Traditional surveys for oil or minerals rely on gravimeters and magnetometers to find hidden deposits. Quantum versions of these instruments massively increase sensitivity. For instance, a quantum gravimeter can detect tiny density variations underground, pinpointing oil reservoirs or mineral veins that classical sensors might miss. By measuring gravitational gradients with cold-atom interferometry, geologists can map cavities or resource-rich ore bodies without drilling, reducing the cost and environmental impact of exploration. In one case, researchers demonstrated that quantum gradiometers could locate underground tunnels and voids by sensing minute gravity changes at the surface. Such precision could improve hydrocarbon prospecting and even help in carbon capture monitoring (ensuring CO₂ stays sequestered in geological formations).
Industrial IoT and Predictive Maintenance – Factories and power plants are full of vibrations, currents, and stresses – and quantum sensors are the ultimate monitors for these signals. Quantum-enhanced accelerometers and magnetic sensors can detect tiny vibrations or magnetic field changes in machinery, forewarning of problems. For example, an array of quantum magnetic sensors could pick up slight changes in the magnetic field of an electric motor that indicate wear in the bearings. Similarly, quantum acoustic sensors might detect microscopic cracks forming in a pipeline by “hearing” frequencies beyond the range of normal microphones. By catching anomalies early, companies can perform maintenance before a catastrophic failure occurs, vastly improving safety and reducing downtime. These sensors would be the backbone of an industrial IoT where AI algorithms constantly analyze the quantum-level data to predict issues – essentially giving each critical machine a “sixth sense” for its health.
Materials Science and Semiconductor Manufacturing – In advanced manufacturing and R&D, knowing what’s happening at the nanoscale is key. Quantum sensing is driving new microscopy and analysis techniques that probe materials non-invasively at atomic scales. One notable example is the use of nitrogen-vacancy (NV) centers in diamonds as nanoscale magnetometers. Companies like Qnami have built quantum microscopes that scan a diamond tip over a microchip to map magnetic fields from individual transistors, helping identify defects and improve chip design. This enables manufacturers to control quality at the level of single atoms – an impossible feat for classical sensors. In material science labs, quantum sensors measure tiny magnetic or electric fields to reveal phenomena like domain structures in ferromagnets or quantum effects in novel materials. Such tools accelerate the development of more efficient batteries, superconductors, and solar cells. In short, quantum sensors act as superpowered eyes and ears on the factory floor and in the lab, leading to better products and more efficient industrial processes.
Fundamental Science: New Eyes on the Universe
Atomic Clocks and Precision Timekeeping – Atomic clocks are often called the ultimate quantum sensors because they measure time by exploiting the quantized energy levels of atoms. Modern optical lattice clocks use lasers tuned to atomic transitions and are so precise, they wouldn’t lose a second over the age of the universe. This mind-boggling precision isn’t just for keeping time; it opens up new scientific frontiers. In 2018, physicists compared two optical clocks with 1×10-18 precision – enough to detect a 1 cm difference in height via gravitational time dilation. In other words, a clock raised just a fingertip higher ticks faster than one below due to general relativity, and quantum timekeeping can measure that! This capability gives rise to “relativistic geodesy,” using clocks to map Earth’s gravitational field more finely than any accelerometer. It also feeds into tests of fundamental physics – probing whether physical constants change over time and enabling tabletop tests of general relativity. Future networks of quantum clocks might even function as massive antennae for gravitational waves or signals of new physics, by detecting the slight ripples in time these phenomena cause.
Detecting Dark Matter and Exotic Physics – Dark matter makes up most of the universe’s mass-energy, yet it has never been directly observed because it interacts so feebly. Quantum sensors are offering clever new ways to hunt for this elusive stuff. In one proposal, scientists use the spins of hyper-polarized helium-3 atoms as quantum detectors for ultralight dark matter particles. The idea is that passing dark matter would nudge the spins ever so slightly, and an exquisitely sensitive quantum spin sensor could register this “tap” where normal detectors see nothing. Likewise, arrays of atomic magnetometers have been deployed in experiments (like the Global Network of Optical Magnetometers, “GNOME”) to search for transient signals that could be from exotic physics or dark matter bursts. Superconducting quantum devices and qubits are also being repurposed as detectors for high-frequency dark matter waves or hidden dimensions. While no dark matter has been found yet, quantum sensing greatly expands the search space, bringing us closer to answering profound questions about the universe.
Gravitational Wave Astronomy & Beyond – When LIGO first detected gravitational waves in 2015, it did so at the very edge of what classical laser interferometers could achieve. To go further, researchers turned to quantum techniques. By injecting squeezed light into LIGO’s detectors – a quantum state of light with lower noise in the measured property – scientists boosted sensitivity and surpassed the “standard quantum limit” of measurement. This upgrade now lets LIGO detect 60% more distant or fainter cosmic events than before. In essence, quantum optics helped “hush” the background quantum noise (the random vacuum fluctuations) that was blurring the measurements. With this enhancement, LIGO and similar observatories can observe more mergers of black holes and neutron stars, opening new windows into the cosmos. Looking ahead, quantum sensors will be central to future gravitational-wave detectors on Earth and in space – whether it’s atom interferometers listening for waves in mid-frequency bands or improved optical cavities using entangled photons as probes. Fundamental science and quantum sensing have a symbiotic relationship: to study the deepest mysteries (origin of the universe, quantum gravity, etc.), we need the most sensitive instruments ever built – and those, inevitably, are quantum in nature.
Conclusion
The few examples highlighted here—ranging from disease diagnostics and cutting-edge medical imaging to advanced defense systems and precision navigation—offer just a small glimpse of quantum sensing’s transformative potential. Even these early applications demonstrate how harnessing quantum phenomena can push measurement sensitivities and accuracies to levels unimaginable with conventional devices.
As engineering hurdles are overcome and fundamental research progresses, we will undoubtedly discover countless additional use cases that tap into this technology’s remarkable capabilities. Scientific exploration will benefit from unprecedented precision—enabling better understanding of complex biological processes, environmental shifts, and possibly even exotic phenomena such as dark matter or new physics. Defense and security solutions will become more reliable and sophisticated, from stealth detection to robust navigation systems immune to external signal interference.
In truth, we are only at the dawn of quantum sensing. Today’s prototypes and initial commercial offerings hint at how deeply this technology could reshape industries and scientific research. As developers learn to exploit the full spectrum of quantum effects—and as manufacturing methods become more refined—we can expect a surge in both incremental improvements and groundbreaking innovations. Put simply, the examples shared represent the tip of an immense iceberg: the true scale and impact of quantum sensing will emerge as its capabilities grow, and with it will come a new era of scientific discovery and strategic advantage.