Quantum Technology Use Cases in Government & Defense
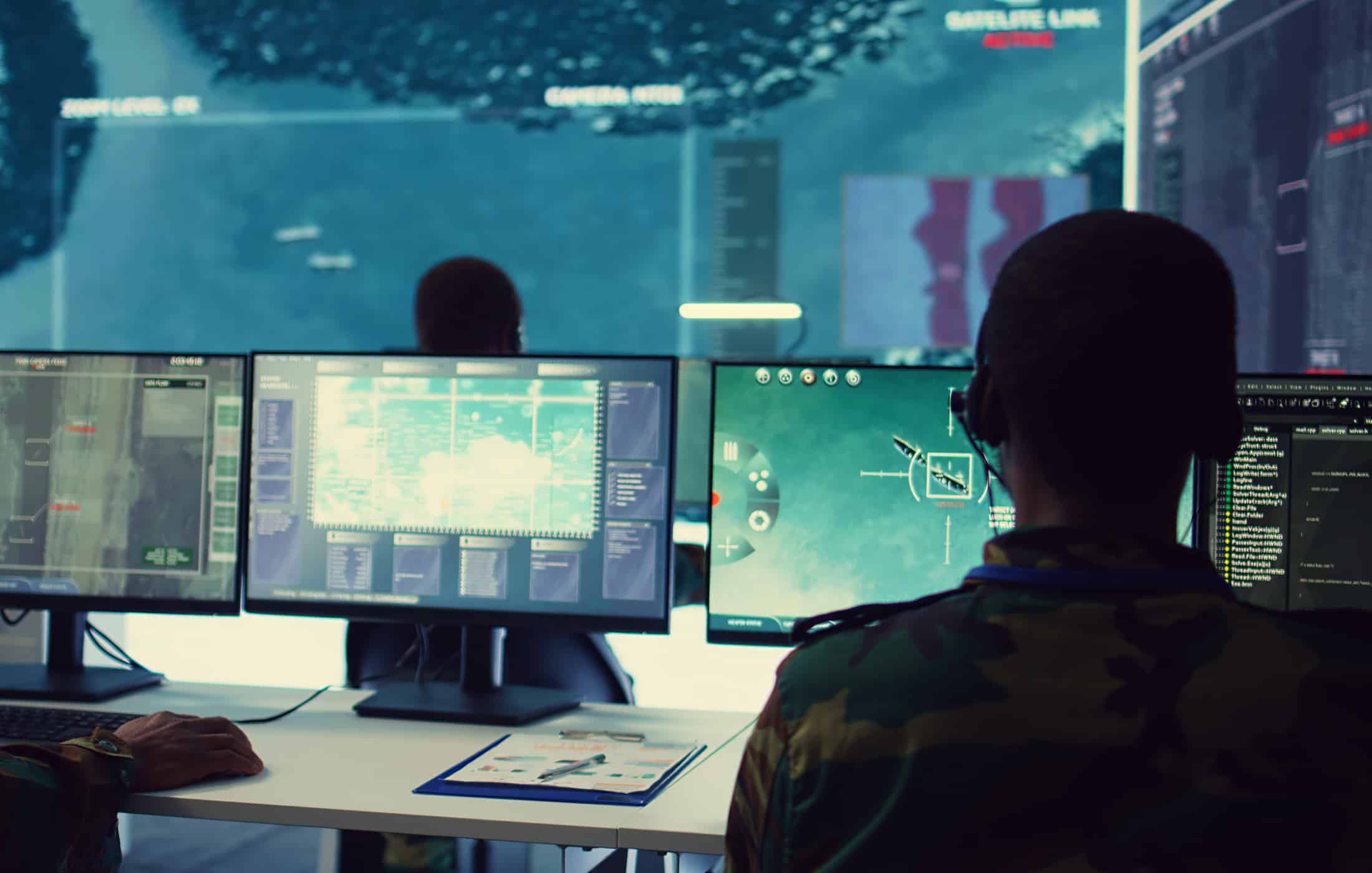
Table of Contents
Introduction
Quantum computing harnesses the counterintuitive principles of quantum mechanics – superposition and entanglement – to process information in ways impossible for classical computers. Unlike binary bits, quantum bits (qubits) can exist in multiple states at once (superposition) and influence each other instantaneously over distance (entanglement), enabling certain computations at exponentially faster scales. These capabilities carry enormous promise for government and defense: solving complex problems in seconds that would take today’s supercomputers millennia, and unlocking new methods for secure communication and sensing. Defense experts warn that the first nation to fully operationalize quantum technology will gain a toolkit of capabilities that could overwhelm unprepared adversaries. Indeed, some analysts compare the current quantum race to the Manhattan Project – a high-stakes technological contest that may determine the strategic balance of the 21st century. In this article, we explore how quantum computing is poised to impact the global government and defense sector – from breakthroughs already underway to the revolutionary changes on the horizon.
Current Developments in Quantum Tech
Quantum computing is rapidly evolving from theory to reality, with government and industry efforts accelerating worldwide.
Research Initiatives: Public-sector investment is at an all-time high. The United States launched a National Quantum Initiative and numerous Department of Defense (DoD) programs, while China’s public spending on quantum tech reportedly exceeds $15 billion – several times the U.S. level. U.S. defense agencies like DARPA are actively partnering with tech companies to benchmark progress. In fact, DARPA’s Quantum Benchmarking Initiative is enlisting multiple firms by 2025 to vet whether their quantum prototypes are on a practical path, with the goal of validating useful defense-ready quantum systems by 2033. Allied governments are also investing: for example, the UK Ministry of Defence recently acquired an on-premises photonic quantum computer to explore data processing for defense applications. This hands-on program with British startup ORCA Computing is helping the UK military gain early experience in quantum machine learning and optimization tasks (like image analysis and decision support) using a compact, room-temperature system.
Public-Private Collaboration: Collaboration between government, academia, and the private sector is seen as crucial to quantum advancement. The U.S. military, for instance, has a history of partnering with industry on emerging tech and is doing so again for quantum. In 2024, the DoD and State of Illinois announced a new Quantum Proving Ground with a $140 million federal investment, creating a shared campus where companies and universities will jointly test quantum prototypes for national security use. Increased funding in the FY2024 defense budget and NDAA is targeting quantum R&D, and the Pentagon has stood up dedicated offices (like the OUSD(R&E) Quantum Science Directorate) to coordinate efforts. Major defense contractors are not standing idle either – many are investing in quantum research or partnering with startups to ensure they aren’t left behind when the technology matures.
Technical Milestones: In the lab, quantum processors are steadily growing in qubit count and stability. Google’s 2019 demonstration of “quantum supremacy” (where a 53-qubit processor solved a problem infeasible for classical supercomputers) was a wake-up call in defense circles. IBM has since unveiled quantum systems with 127 and 433 qubits, on roadmaps aiming for thousand-qubit devices in the next couple of years. While these noisy intermediate-scale quantum (NISQ) devices can’t yet outperform classical computers on most real-world tasks, they prove rapid progress. Key defense-related applications like quantum encryption and sensing have already moved from theory toward field tests. For example, quantum navigation systems to counter GPS jamming are being prototyped by the Defense Innovation Unit and others. Early quantum-secure communication networks are also in trial: China demonstrated a 4,600 km quantum key distribution network integrating satellites and fiber lines, and the EU and US are funding quantum internet testbeds. In short, governments recognize that preparing for quantum’s disruptive potential is no longer optional – it’s imperative for future security.
Industry-Specific Use Cases
Quantum computing’s most significant impacts for defense and government are expected in a few key domains. Below we break down these use cases – from code-breaking to sensors – and how quantum advantages could be applied in each area.
Cryptanalysis and Post-Quantum Cryptography
One of the most widely discussed defense implications of quantum computing is its ability to break current encryption, threatening the foundations of cybersecurity. Cryptanalysis: Today’s public-key encryption (used in everything from military communications to online banking) relies on mathematical problems that are practically impossible for classical computers to solve in reasonable time – for example, factoring large numbers underpins RSA encryption. Quantum algorithms change that calculus. In 1994, mathematician Peter Shor discovered a quantum algorithm that can factor large numbers exponentially faster. In theory, a full-scale quantum computer running Shor’s algorithm could crack a 2048-bit RSA key – something that would take a classical machine trillions of years – in a matter of hour. It could similarly break other asymmetric ciphers like elliptic-curve cryptography. This means that a nation with a powerful quantum computer could potentially decrypt an adversary’s sensitive communications, exposing military secrets and state communications that were assumed secure.
Fortunately, such capabilities remain largely future tense – by most estimates, the error-corrected quantum computers needed to attack modern RSA are still a decade or so away. However, the threat isn’t theoretical for defense planners. Adversaries could be stockpiling encrypted intercepts now, planning to decrypt them once quantum hardware is available – a strategy known as “harvest now, decrypt later.” Classified government data that needs to remain secret for decades is particularly at risk.
To get ahead of this threat, the security community is racing to deploy post-quantum cryptography (PQC): new encryption algorithms designed to resist quantum attacks. The U.S. National Security Agency has mandated a transition of its systems to quantum-resistant algorithms by 2035, and NATO and other governments are following suit. The U.S. National Institute of Standards and Technology (NIST) has already selected a suite of PQC algorithms for standardization, paving the way for wider adoption. This transition is a massive undertaking – essentially upgrading the cryptographic infrastructure of the entire government and defense enterprise. Pentagon cybersecurity officials have made it a top priority, urging a proactive review of all encryption in use and collaboration with vendors to swap in quantum-safe solutions before it’s too late. In the meantime, intelligence agencies are also exploring quantum computing for their own offensive cyber operations – hoping to wield the code-breaking power of quantum before adversaries do. The stakes are high: a fully developed quantum computer could render much of today’s encrypted government data transparent, unless robust countermeasures are in place.
Secure Communications and Quantum Networking
If quantum computing threatens encryption, it also offers new tools to communicate securely. Quantum Key Distribution (QKD): QKD is a method of securely exchanging encryption keys using quantum signals, and it promises information-theoretic security – meaning even an eavesdropper with unlimited computing power (yes, even a quantum computer) cannot intercept the key without detection. QKD leverages the no-cloning theorem of quantum physics, which says quantum states (like a photon’s polarization carrying a bit of key) cannot be copied perfectly. Any interception disturbs the quantum state and reveals the presence of an eavesdropper by causing detectable errors in the key exchange. For defense communications – which must often travel over insecure or contested channels – this is a game changer. Imagine command orders or intelligence data links that you know cannot be secretly listened to by the enemy because physics itself prevents it. China has heavily invested in QKD, launching the world’s first quantum communications satellite and building an integrated space-to-ground QKD network spanning thousands of kilometers. They reportedly achieved key distribution rates over 100 Mbps, a record for quantum comms. Several European nations and Japan have also fielded QKD test networks. However, practical challenges remain: QKD distance is limited by signal loss in fibers or air, and existing systems can be slow (current tech transmits on the order of megabits per second). There are also implementation vulnerabilities (e.g. side-channel attacks on detectors) that require careful protocol design. Due to these limitations, U.S. agencies like the NSA have so far expressed skepticism about deploying QKD for national security needs until it matures.
Beyond QKD, the long-term vision is a quantum network or “quantum internet” that could connect quantum devices (sensors and computers) across the globe. In theory, a network of quantum computers could share entanglement and tackle problems collectively that no single quantum processor could solve. For defense, such a network could enable distributed quantum sensing (e.g. arrays of quantum detectors acting in unison) and ultra-secure multi-site communications. Governments are pouring research dollars into quantum repeaters – devices needed to extend quantum links over long distances by swapping entanglement without violating no-cloning rules. Recent experiments have shown progress toward entanglement swapping and memory-free quantum repeaters. While a full-blown quantum internet is likely still many years away, incremental steps like secure quantum networks for military bases or ships are closer. In the near term, we may see defense networks combining classical channels (for bulk data) with QKD-secured links for encryption keys, ensuring that even if adversaries tap the fiber, they can’t undetectably steal the keys. Ultimately, as quantum computing advances, quantum-safe and quantum-based communications will evolve in tandem – with post-quantum classical encryption and quantum link security working together to protect government information. The end goal is to outpace adversaries so that allied forces can communicate with absolute confidentiality, even in the face of quantum-enabled enemies.
Optimization of Logistics and Operations
Modern militaries and governments grapple with enormous optimization challenges – from efficiently routing convoys and scheduling maintenance, to allocating limited resources across dynamic battlefields. Many of these problems are combinatorially complex, meaning the number of possible solutions is astronomically high and grows explosively with the problem size. Quantum computers have shown promise for certain optimization tasks by exploiting quantum parallelism. While today’s known quantum optimization algorithms usually offer more modest polynomial speedups (as opposed to exponential), even these improvements could be significant in practice. For instance, if a classical system takes 10,000 hours (~14 months) to compute an optimal logistics plan for a large-scale deployment, a quantum optimizer with a polynomial speedup might cut that to 100 hours (a few days). That difference could translate to major operational gains – faster routing of supplies, quicker re-planning when conditions change, or more efficient deployment of assets, all of which can be decisive in conflict or crisis.
Defense analysts have identified a plethora of relevant optimization use cases: optimizing transportation and supply chains, scheduling surveillance or satellite coverage, devising flight plans for multi-drone swarms, assigning targets and routes to squadrons of strike aircraft, configuring cyber defenses dynamically, and solving the “knapsack” problems of allocating weight and power on platforms. Even missile defense – deciding how to pair interceptors with incoming targets in real time – is fundamentally an optimization problem. Quantum computers (and even intermediate quantum-inspired algorithms) are being explored to tackle these challenges. In fact, the U.S. DARPA has run programs in quantum-inspired optimization to see if the unique approaches of quantum computing can yield better classical algorithms as a stepping stone. Early results indicate that new heuristics can outperform existing methods for certain defense problems by orders of magnitude. Already, some defense contractors have tested quantum annealing machines (a form of quantum optimizer) for things like flight scheduling and radar waveform design, though with mixed results so far. The consensus is that as quantum hardware improves, so will its edge on these complex planning tasks. Even a 10× speedup means solutions in hours instead of days – enabling a military to act inside an adversary’s decision loop. Quantum optimization could thus enhance operational agility, allowing warfighters and analysts to evaluate many more scenarios and courses of action under tight time constraints. In sum, while quantum won’t instantly solve all hard optimization problems, it offers a powerful new approach to crunch through the defense logistics “to-do list” faster and more efficiently.
Artificial Intelligence and Machine Learning
Artificial intelligence is already transforming defense by enabling capabilities like autonomous drones, rapid image analysis, predictive maintenance, and decision support systems. Training these AI systems, however, often demands massive computing resources and datasets. This is an area quantum computing might eventually turbocharge. Quantum Machine Learning (QML): Researchers have theorized quantum algorithms that could accelerate machine learning tasks – for example, finding patterns in large datasets or training deep learning models – by taking advantage of high-dimensional quantum state spaces. Certain problems might see exponential speedups, while others get polynomial improvements, but even the possibility is enticing for data-intensive defense missions. Imagine swiftly analyzing thousands of hours of surveillance footage or training a battlefield AI model on-the-fly to recognize new threats – tasks that currently might be limited by classical processing speeds. Quantum algorithms like the Harrow-Hassidim-Lloyd (HHL) algorithm (for solving linear systems) or variational quantum classifiers are being studied for applications in pattern recognition, logistics forecasting, and sensor data fusion.
That said, most QML algorithms are still theoretical or in early research, and today’s quantum computers are too small and error-prone to outperform classical AI hardware. Challenges include how to efficiently encode real-world data into quantum states and how to handle noise and errors that can derail learning algorithms. However, the defense community is preparing now by experimenting with hybrid approaches. Notably, the UK’s MoD, using the ORCA photonic quantum computer, is exploring machine learning tasks like image and handwriting recognition on quantum hardware. The goal is to understand how a quantum co-processor might assist in analyzing reconnaissance imagery or decrypting signals faster than a classical computer alone. Results from that program will inform future AI development. In the long term, if large-scale quantum computers become available, they could dramatically speed up AI model training and optimization, enabling more capable AI for defense. Complex models that today require specialized supercomputers (or large GPU farms) and weeks of training might be trained in hours on a quantum system. This could put advanced AI into the field faster – for example, allowing real-time re-training of algorithms to adapt to new enemy tactics or electronic warfare measures. Moreover, quantum computers might handle massive sensor data streams better, finding subtle correlations or anomalies (a key aspect of intelligence analysis and threat detection) that classical analytics miss. In summary, quantum computing and AI have a symbiotic potential: quantum can supercharge AI, and AI can help manage and program quantum systems. The defense sector is keenly watching this intersection, as it could yield smarter autonomous systems and decision aids that maintain an edge over adversaries.
Quantum-Enhanced Simulations
Simulation is arguably the most practical near-term application of quantum computing. Governments and defense agencies rely heavily on simulations – whether it’s modeling new materials for armor and aircraft, testing nuclear reactions, or war-gaming different conflict scenarios. Classical computing has limits in simulating quantum-mechanical systems, in particular. Materials and Chemistry: Quantum computers excel at simulating other quantum systems, because qubits themselves obey quantum rules. This could be transformative for materials science and drug development. In a defense context, one example is high-temperature superconductors – materials that conduct electricity with zero resistance. Such materials could revolutionize naval propulsion, sensor systems, and energy storage if found to work at ambient temperatures. Today, discovering new superconducting materials is largely trial-and-error because the quantum interactions in candidate compounds are too complex for classical supercomputers to simulate exactly. Quantum computers could model these interactions directly, potentially unraveling the physics needed to engineer superconductors that function in battlefield conditions. The same goes for advanced battery chemistries, stealth coatings, or explosives – any technology where quantum-scale interactions matter (molecule bonding, electron behavior) might be better understood and optimized via quantum simulation. Researchers have noted that quantum simulation could lead to lighter, stronger, and more efficient materials for the military, giving everything from soldiers’ gear to spacecraft improved performance.
Beyond materials, quantum simulation can aid in strategic domains. For instance, simulating complex chemical/biological processes can help in developing countermeasures to weapons of mass destruction or new medical treatments for troops. Simulating quantum physics is also directly relevant to designing quantum sensors and quantum communication components – essentially using a quantum computer to help build other quantum tech. Even in climate and space modeling, quantum simulations could refine predictions (useful for planning operations impacted by weather or solar activity). It’s important to note that quantum simulators don’t need to be universal quantum computers; special-purpose quantum devices might solve specific simulation tasks sooner. National labs and defense research offices are already using early quantum machines to study simple chemical reactions and materials. Over time, these efforts will expand. The big-picture benefit is that better simulations lead to better designs and strategies. Whether it’s a more effective polymer for body armor or a precisely engineered alloy for hypersonic missiles, quantum computing may significantly cut down the R&D timeline by letting scientists test ideas virtually with high fidelity. As Nobel laureate Richard Feynman, who first proposed quantum computing, envisioned, using quantum machines to simulate quantum physics could unlock innovations that classical computers would never find. Defense innovation could accelerate, giving early adopters a technological edge.
Space and Satellite Applications
Space is both an opportunity and a vulnerability for modern defense. Quantum technologies are finding their way into space for secure communications and enhanced sensing. Secure Satcom and Quantum Satellites: Satellites play a crucial role in military communications, and researchers are demonstrating quantum encryption over satellite links. In 2017, China’s Micius satellite enabled the world’s first intercontinental quantum-encrypted video call, showcasing how QKD could be extended to distant sites via space. Since then, China has integrated satellites into a national quantum communication network, and Europe is planning a “EuroQCI” quantum communications constellation. By using satellites as relay nodes for QKD, one can securely connect government facilities across the globe, as photons carrying quantum keys are exchanged between ground stations and satellites in orbit. Space-based quantum links help overcome the distance limits of ground fiber (since most of the path is vacuum with minimal loss). The defense implication is a future where communications with overseas bases, ships at sea, or even forward-deployed units can be secured by quantum keys delivered via satellite, immune to interception. There are challenges in making these systems robust – satellite payloads must protect fragile quantum sources and detectors against vibration and radiation – but steady progress is being made. Governments are already testing quantum communications with satellites and high-altitude UAVs, aiming to create an ultra-secure “quantum intranet” for the military.
Quantum Sensing from Orbit: Satellites have long been eyes in the sky. Quantum sensors could make them even more discerning. Highly sensitive magnetometers and gravimeters based on quantum effects can detect tiny variations in Earth’s magnetic and gravitational fields. These variations can betray the presence of hidden objects or subterranean structures. For example, a quantum gravimeter in orbit or on an aircraft might spot the slight gravitational anomaly caused by a submerged submarine or an underground bunker due to the mass difference with the surrounding environment. Quantum sensors can also improve navigation and timing in space. The GPS satellites themselves carry atomic clocks (an early quantum technology) for precise timing; future navigation satellites might use even more precise quantum clocks (like optical lattice clocks) to improve positioning accuracy for everyone. Additionally, space-based quantum sensors could enhance ISR (intelligence, surveillance, reconnaissance) capabilities. The U.S. military is interested in integrating quantum sensors into space platforms to detect threats like nuclear materials or to enable navigation in GPS-denied scenarios. In one notable program, DARPA’s Robust Quantum Sensing (RoQS) initiative is developing rugged quantum sensors for deployment on satellites and aircraft, aiming to maintain performance even under the harsh conditions of launch and maneuver. The ability to passively sniff out electromagnetic or gravimetric anomalies from space, without active signals, could provide invaluable strategic intelligence.
Finally, space is an excellent environment for fundamental quantum experiments (microgravity can extend coherence times for quantum states). Countries like China, Canada, and Italy have run quantum experiments on orbit to inform future tech. All these efforts point toward a convergence of quantum tech and the space domain. In the coming years, we can expect defense satellites that not only broadcast navigation signals and take pictures, but also exchange entangled photons, run quantum encryption protocols, and host exotic sensors. Space-based quantum assets will augment terrestrial networks, creating a multilayered, hard-to-intercept communication and sensing grid for militaries. As an example of the direction: China claims to be exploring quantum radar satellites that could detect stealth aircraft from orbit by using entangled microwave signals – though such claims remain unproven and met with skepticism. Whether or not quantum radar becomes reality, it’s clear that quantum tech aloft will play a growing role in surveillance and secure command-and-control links.
Quantum Sensing for Detection, Navigation, and Stealth
Quantum sensing applies the extreme sensitivity of quantum systems to measure physical quantities – and it has some of the most immediately promising defense applications. Intriguingly, the same fragility that makes qubits hard to control in a computer (they decohere from slightest disturbances) can be useful in sensors: a qubit makes an excellent probe for changes in its environment.
Inertial Navigation: One high-priority application is quantum inertial navigation. Today’s submarines, ships, and aircraft use inertial measurement units (gyroscopes and accelerometers) to track their movement when GPS signals are unavailable or jammed. But even the best classical inertial systems drift over time, accumulating error. A state-of-the-art naval gyro might drift by over a kilometer per day, which is why GPS updates are needed regularly. Quantum accelerometers and gyros, leveraging techniques like atom interferometry, promise orders of magnitude greater precision. In recent tests, a hybrid quantum accelerometer achieved 50× better stability than the best classical devices. Such a sensor could enable a submarine to navigate silently for long periods with minimal position error, or an aircraft to hold course in a GPS-denied conflict zone without constant corrections. For the military, this means a backup navigation method that is immune to GPS jamming and spoofing – a game-changer for resiliency. While quantum inertial units are still in development (the challenge is to make them small, fast, and robust outside the lab), the promise of “GPS-free navigation” has driven significant R&D. The U.S., U.K., and China have all demonstrated early prototypes. In the coming years, we may see high-end naval vessels and strategic aircraft equipped with quantum INS systems as a hedge against satellites being knocked out or spoofed.
Stealth Detection and Quantum Radar: Quantum sensing could also pierce modern stealth technology. Stealth aircraft are designed to evade conventional radar by minimizing radar signal returns. Quantum radar concepts seek to use entanglement or other quantum phenomena to detect objects with much higher sensitivity and perhaps even identify unique “quantum signatures.” Chinese researchers have claimed breakthroughs in a quantum radar that could detect stealth planes at 100 km range by using entangled photons. The idea is that entanglement between transmitted and reference photons could reveal the presence of objects that would otherwise scatter signals below the noise floor. If those claims hold (no independent verification yet), it would potentially neutralize billions of dollars worth of stealth technology. Western scientists remain cautious – building a practical quantum radar is immensely difficult – but defense labs in the U.S. and Europe are certainly investigating these techniques. Even if true quantum radar proves elusive, other quantum sensors can aid in detection. Quantum magnetometers are so sensitive that they might detect the magnetic field disturbances of a submerged submarine or a hidden vehicle’s wiring. Quantum RF receivers (like Rydberg atom sensors) can pick up a broad spectrum of radio emissions with tiny antennas, potentially improving electronic intelligence and spectrum awareness. And quantum lidar systems could improve detection of objects with low reflectivity or camouflaged targets by using squeezed light or single-photon detection techniques beyond classical limits.
In summary, quantum sensing offers enhanced eyes and ears for the military: from finding the proverbial needle in a haystack (a stealthy threat in vast noise) to providing precise navigation when GPS is dark. Some quantum sensor technologies (high-precision atomic clocks and magnetometers) are already fielded in niche roles. Others (like quantum inertial nav and exotic radar) are in prototypes and experiments. As they mature, expect to see them integrated into platforms: jets with quantum-enhanced navigation units, ISR aircraft carrying quantum detection pods, even infantry units equipped with quantum gravimeters to detect tunnels or underground caches. And notably, these sensors tend to passively detect or navigate without emitting telltale signals, which is a huge tactical advantage. A quantum navigation system, for example, doesn’t need to radiate anything externally – it just measures internal quantum states – so it cannot be jammed or detected by an adversary. This “silent” operational mode fits perfectly into the needs of stealth operations and covert surveillance. Quantum sensing is thus poised to augment the situational awareness and survivability of defense assets in significant ways.
The Arrival of Universal Quantum Computing
What happens when fully universal, fault-tolerant quantum computers finally arrive? The consensus is that we will see both disruptive threats and powerful benefits for the government and defense sector. On the disruptive side, a universal quantum computer – one with thousands or millions of stable qubits, capable of running any algorithm reliably – would render many current cryptographic tools obsolete. As discussed, public-key encryption (RSA, ECC) would be broken. This raises the alarming prospect that an adversary with such a machine could read every secret of a nation still using old encryption – from diplomatic cables to weapons blueprints. Intelligence agencies are acutely aware of this “Q-day” scenario. It’s why there is urgency to switch to post-quantum encryption well before large quantum computers are operational. A less obvious threat is to blockchain and authentication systems; blockchains (used in everything from cryptocurrency to logistics) could be forged by quantum attackers, and digital signatures (which secure software updates, documents, etc.) could be falsified. Essentially, a universal quantum computer could undermine the integrity of digital systems across military and civilian infrastructure if defenses aren’t in place. Governments are planning for this by inventorying vulnerabilities and establishing quantum-readiness roadmaps now.
There’s also a geopolitical destabilization risk if one nation achieves large-scale quantum computing significantly ahead of others. It could secretly decrypt others’ communications (leading to strategic advantages or crises), or even threaten economic systems by cracking financial encryption. This asymmetry could tempt preemptive actions. Some analysts worry about a quantum-powered intelligence coup analogous to the Allies’ Ultra secret in WWII (where breaking Enigma codes yielded immense advantage) but on a far grander scale. It might also spur nations to react rashly if they suspect their nuclear command-and-control could be compromised by quantum decryption. Such scenarios underscore the need for international dialogue on responsible use of quantum computing, much as was done for nuclear weapons. There may emerge treaties or norms against using quantum computers for certain offensive cyber operations – though verification would be difficult.
On the positive side, universal quantum computers would be a boon to defense science and engineering. Many of the nascent applications we discussed (optimization, AI, simulation) would fully blossom. Any computational problem that currently taxes supercomputers might be solvable in a heartbeat. For example, complex war-game simulations that involve myriad variables and stochastic elements could be run at ultra-high fidelity, allowing planners to explore outcomes in a way never possible before. Material and drug discovery would accelerate dramatically – perhaps yielding new medicines to protect soldiers from bioweapons, or new metamaterials that give aircraft invisibility to conventional sensors (ironic, as quantum might help counter stealth but also create new stealth). Logistics and autonomous systems would also benefit: a universal quantum AI could coordinate vast numbers of assets (drones, satellites, ground units) in real time, finding optimal strategies on the fly. Even mundane tasks like personnel scheduling or efficient budgeting across defense projects could be optimized to perfection.
In the intelligence realm, fully developed quantum computers could break not only codes but also solve complex analytic problems. They might crack difficult puzzles like optimizing supply routes under enemy disruption (a variant of the traveling salesman problem) or figuring out molecular signatures of nuclear materials from limited sensor data. Quantum algorithms might sift through enormous data lakes of intercepted signals or open-source intelligence and pull out patterns undetectable to classical analytics, giving early warning of threats. In space, quantum computers on satellites could autonomously manage swarms of satellites or process imagery with unprecedented speed right on orbit.
There will also be unexpected innovations – as one expert noted, the “toolkit” of capabilities from quantum will let its possessor shape the world in ways we can barely imagine today. We might see entirely new paradigms of conflict (perhaps quantum vs quantum, where competing quantum AIs or quantum encryption systems duel each other). Warfare could extend into the quantum domain, with states trying to disrupt each other’s quantum infrastructure or steal qubit technology. On the defensive side, fully mature quantum computers might enable unbreakable authentication codes (using quantum one-time pads) or ultra-secure clouds for storing data.
However, getting to this point won’t be easy. Current estimates suggest it may take 20–30 years of sustained progress to build scalable, error-corrected quantum computers that can reliably run long algorithms. The engineering challenges (isolating thousands of qubits from noise, implementing error correction with millions of physical qubits) are monumental. But the trajectory is positive, and every year brings new records in qubit count and coherence time. Governments are actively monitoring milestones – for instance, if someone demonstrates a quantum computer breaking a RSA-2048 in the lab, that will send shockwaves through national security establishments (and likely spur crash programs to upgrade encryption immediately). In summary, the arrival of universal quantum computing will be a watershed moment. It stands to upend many aspects of cybersecurity and computation that defense organizations take for granted, even as it equips those same organizations with extraordinary new capabilities. The prudent course is to anticipate and prepare for both outcomes: mitigate the risks and exploit the benefits. As one DoD official put it, you don’t want to be the side still “playing defense” when the quantum breakthrough occurs.
Sector Preparation and Responses
Recognizing the quantum future, governments, militaries, and defense contractors worldwide are taking proactive steps to prepare. National Strategies and Investment: Over 17 countries have published national quantum technology strategies, many emphasizing security applications. The United States’ approach includes hefty funding for research (over $800 million annually across DoE, NSF, DoD, etc.), the establishment of quantum research centers, and legislation like the National Quantum Initiative Act to coordinate efforts. The EU launched a €1 billion Quantum Flagship program, and individual European nations have their own defense-oriented quantum projects. China’s “whole-of-nation” effort – building national labs, pumping resources into education, and incentivizing industry – is explicitly aimed at leapfrogging the West in quantum computing and cryptography. In response, the U.S. and allies are boosting collaboration: joint research projects, information-sharing on quantum threats, and even discussions of export controls on critical quantum technologies to prevent them from flowing to adversaries. A CSIS analysis noted that allied cooperation is a huge asset; many top quantum researchers are in the U.S. or partner countries, so pooling talent and establishing common standards can help democratic nations stay ahead. There’s also an emphasis on workforce development – growing the next generation of quantum engineers and scientists. Governments are investing in STEM education and specialized quantum training programs to ensure they have the human capital for both research and the practical implementation of quantum systems. This is critical: having a cutting-edge quantum computer is useless without experts who know how to apply it to defense problems.
Military and Agency Initiatives: Within defense departments, specialized teams are evaluating quantum tech and beginning integration where feasible. For example, the U.S. Army designated a Quantum Research Center to coordinate quantum information science efforts across its research labs. The Navy’s Information Warfare Center (NIWC Pacific) has a “Quantum Advantage” project to train personnel and prototype quantum applications for naval use. Across the Pentagon, quantum working groups are identifying which systems need upgrading to PQC, running war-game scenarios of a “quantum-enabled adversary,” and funding pilot projects. The DoD’s Defense Innovation Unit (DIU) has already solicited commercial solutions for quantum sensing and is test-running them in real military environments. DARPA, known for its forward-looking R&D, is not just funding long-range research but also acting as an independent tester of industry claims (through programs like QBI) to avoid being duped by hype. This shows a healthy skepticism: defense agencies want to see real performance, not just promises, before deploying quantum tech. At the national security agencies (NSA, CIA, etc.), much of the preparation is classified, but one can assume heavy focus on cryptography and signals intelligence – ensuring that when “Q-Day” arrives, their secrets are safe and, conversely, that they can exploit others’ vulnerabilities. NSA’s public statements make clear they intend to have quantum-resistant cryptography in place across U.S. national security systems well before practical quantum code-breaking is possible.
Defense contractors and the industrial base are likewise positioning themselves. Major firms like Lockheed Martin, Northrop Grumman, and Boeing have internal quantum research groups or investments in quantum startups. They are exploring how quantum computing might optimize complex engineering designs or improve C4ISR systems. Some have even bought early quantum hardware for testing algorithms. This mirrors the early days of classical computing, where industry doesn’t want to be left without in-house expertise once the technology matures. Additionally, contractors are partnering with governments on prototype projects – for instance, providing platforms to host quantum sensors or integrating quantum encryption into existing comms systems to evaluate compatibility. The goal is to shorten the adoption curve: if quantum tech suddenly hits maturity, the defense sector wants to plug it in quickly rather than start from scratch. We see a precedent in how AI was rapidly adopted; those who had done pilot studies in advance could operationalize AI faster when it became viable. The same is likely with quantum.
Policy and Regulation: Governments are also crafting policy frameworks for quantum tech. This includes funding incentives (grants, challenges) to spur innovation in the private sector, as well as considering regulatory measures. Export controls are one area: there’s discussion about controlling the sale of advanced quantum cryptography systems or certain quantum manufacturing equipment, to prevent them from reaching hostile actors. Patent filings in quantum tech are skyrocketing, raising intellectual property questions – nations want to ensure they secure key patents, or at least access to them, for national security uses. Standard-setting is another preparatory action: NIST and other bodies are working on standards for PQC, quantum random number generators, and QKD protocols so that when agencies buy these products, they meet security requirements. Even legal frameworks are being looked at – for example, how to certify a encryption method as quantum-resistant, or how to manage liability if someone’s algorithm that was thought secure gets cracked by a quantum attack. In essence, the defense-industrial complex is gearing up now so that quantum technologies can be transitioned out of labs and into real deployments as smoothly as possible. There’s a recognition that we can’t wait until a breakthrough happens to start figuring out the logistics. By investing early – in people, partnerships, and plans – governments hope to shorten the gap between quantum invention and military impact.
Challenges and Risks Ahead
Despite the enthusiasm, significant challenges and risks accompany the quest for quantum computing in defense.
Technical Hurdles: The first challenge is simply making quantum computers that are powerful and reliable enough for real-world use. Qubits are extraordinarily sensitive – stray heat, electromagnetic noise, or fabrication defects can cause errors (decoherence) that crash computations. Engineering scalable machines means finding ways to correct these errors faster than they occur. Quantum error correction requires a huge overhead: dozens or hundreds of physical qubits just to safeguard a single “logical” qubit of high-fidelity information. This means a supposedly “million-qubit” computer might effectively only have a few thousand robust qubits after error correction. Progress is being made (labs have demonstrated error correction on small scales), but it’s a long road, and some in the field caution that timelines for a fault-tolerant quantum computer are still very uncertain. For defense planners, this raises the question of how to prioritize resources: invest too early, and one might pour money into technology that isn’t battle-ready for years; invest too late, and you risk strategic surprise. Managing expectations and sustaining funding for a long-term R&D effort (with perhaps slow, incremental payoffs) is a non-trivial challenge in the government budgeting process, which often prefers quick wins.
Hype vs Reality: Relatedly, there is a hype risk. Quantum computing has gained buzzword status, and not every claim holds up. Some companies have, in the past, exaggerated quantum capabilities to secure contracts or investments. The defense sector must remain vigilant against “quantum snake oil.” This is partly why DARPA’s approach of rigorous benchmarking is important – to separate real progress from vaporware. If expectations aren’t managed, there’s a risk of a hype boom-bust cycle that could sour political and public support for quantum research (much like AI went through winters). The ethical use of funds is also a consideration: billions are being spent globally, so transparency and international peer review of claims help ensure money is well-spent on genuine advances.
Security Risks: On the flip side, if and when quantum capabilities do come to fruition, they introduce new security risks. A big one we discussed is the threat to encryption – which not only affects military comms but civilian infrastructure and the global financial system. Governments worry about a “cryptopocalypse” where a lot of currently secure data suddenly becomes readable. This extends beyond secrets: think of the trillions of dollars in e-commerce and banking transactions protected by encryption daily. A collapse of digital trust due to quantum cracking could have massive economic and social consequences. Even before that point, the fear of quantum espionage might erode trust in communications. We may see geopolitical tension as nations suspect each other of eavesdropping via quantum – even, perhaps, accusing one another of decrypting diplomatic messages and acting on that intel. Another risk is that quantum computers could be turned to offensive cyber operations beyond cryptography – for example, quickly solving certain hard computational problems to optimize cyberattacks or malware. While classical computing is more than sufficient for most hacking, a quantum edge might enable new tactics.
Geopolitical Competition and Inequality: The race to achieve quantum supremacy (in the sense of practical dominance) has been likened to a new arms race. This competition can be risky if it leads to unilateral actions. If one country, say, is clearly pulling ahead, others might panic or engage in espionage to catch up – indeed, there have already been reports of hacking groups targeting quantum research labs to steal IP. There’s also the concern that quantum advantage might be unevenly distributed globally, potentially widening the power gap. If only a few superpowers control the majority of quantum computing resources, it could leave smaller nations or international institutions at a disadvantage in cybersecurity and technological development. This could upset global stability or drive non-holders to form coalitions and resource-sharing agreements (much as countries without advanced spy satellites collaborate to access imagery).
Conversely, if quantum tech proliferates widely (like classical computing eventually did), there’s the risk of it falling into the hands of rogue actors. Today, building a quantum computer is far beyond the capability of terrorist or criminal groups. But in a couple of decades, if modular quantum computers can be bought or built from kits, sophisticated criminal networks might obtain them. They could use them to crack encrypted files (perhaps stealing state secrets or corporate intellectual property) or to undermine blockchain-based systems that underpin cryptocurrencies (potentially to steal funds). This means law enforcement and counterintelligence might face quantum-empowered adversaries in the future, complicating an already challenging threat environment.
Ethical and Legal Concerns: The introduction of powerful quantum tech also raises new ethical questions. For example, ultra-precise quantum sensors could enable forms of mass surveillance that test legal boundaries. If you can passively detect devices or people through walls via quantum sensing, how does that square with privacy rights? Military use of such sensors in war is one thing, but their use domestically or by intelligence agencies will require policies to prevent abuse. Similarly, if quantum computers break encryption, there’s an ethical line regarding to what extent governments should exploit that. In liberal democracies, there will be debates about law enforcement access to encrypted communications – quantum could either be seen as a threat to privacy (if governments can read anything) or a necessary tool to stop criminals who “go dark.” The technology might force a reexamination of surveillance laws and norms.
International law will also need to catch up: Is hacking another country’s systems using a quantum computer considered an armed attack or an intelligence activity? Can quantum algorithms be considered a weapon (the way we consider cyber weapons)? These are uncharted waters. And finally, there’s a risk of strategic miscalculation. If Country A suddenly achieves a big quantum breakthrough, Country B might fear a “quantum Pearl Harbor” and react aggressively. Lack of transparency – quantum work is often shrouded in secrecy – could lead to worst-case assumptions. Confidence-building measures, perhaps sharing some information on quantum progress in international forums, might be needed to avoid an atmosphere of paranoia.
In short, while quantum computing holds incredible promise, defense and government leaders must navigate a minefield of challenges to get there. They need to continue pushing the science forward (so as not to fall behind adversaries), and simultaneously harden their security posture against quantum threats. They must hype the promise to secure funding, and temper that hype with realism so that efforts are sustained. Policies must be crafted in advance of the tech’s arrival, an unusual predicament given how unpredictable the timeline can be. As the Marine Corps University aptly noted, most quantum technologies of this “second quantum revolution” are still decades from deployment, yet failing to prepare for them would be a grave mistake. Balancing present readiness with future preparedness is the name of the game.
Conclusion
Quantum computing is on the cusp of reshaping government and defense, much as radar or the internet did in earlier eras. It promises enhancements across the board – unbreakable communications, unprecedented computing power for logistics and AI, new sensors that reveal hidden threats, and simulations that accelerate innovation. It also carries profound disruptive potential, especially for cybersecurity, meaning it can just as easily undermine a nation that is unprepared. The coming decades will likely see a race between quantum offense and defense: code-makers vs code-breakers, detection vs stealth, and those who harness quantum vs those who do not. Governments around the world have recognized this and started investing in research, talent, and protective measures to ensure they stay on the right side of that equation.
In the end, quantum computing in government and defense is not about any single application – it’s about a paradigm shift in information power. From codebreaking to navigation, from strategic planning to real-time battlefield decisions, the advantage will go to those who can leverage computing might that we once thought impossible. We stand at the beginning of this quantum journey. The next few years will reveal which breakthroughs happen faster than expected and which challenges prove harder. For now, the course is set.