Transmon Qubits 101
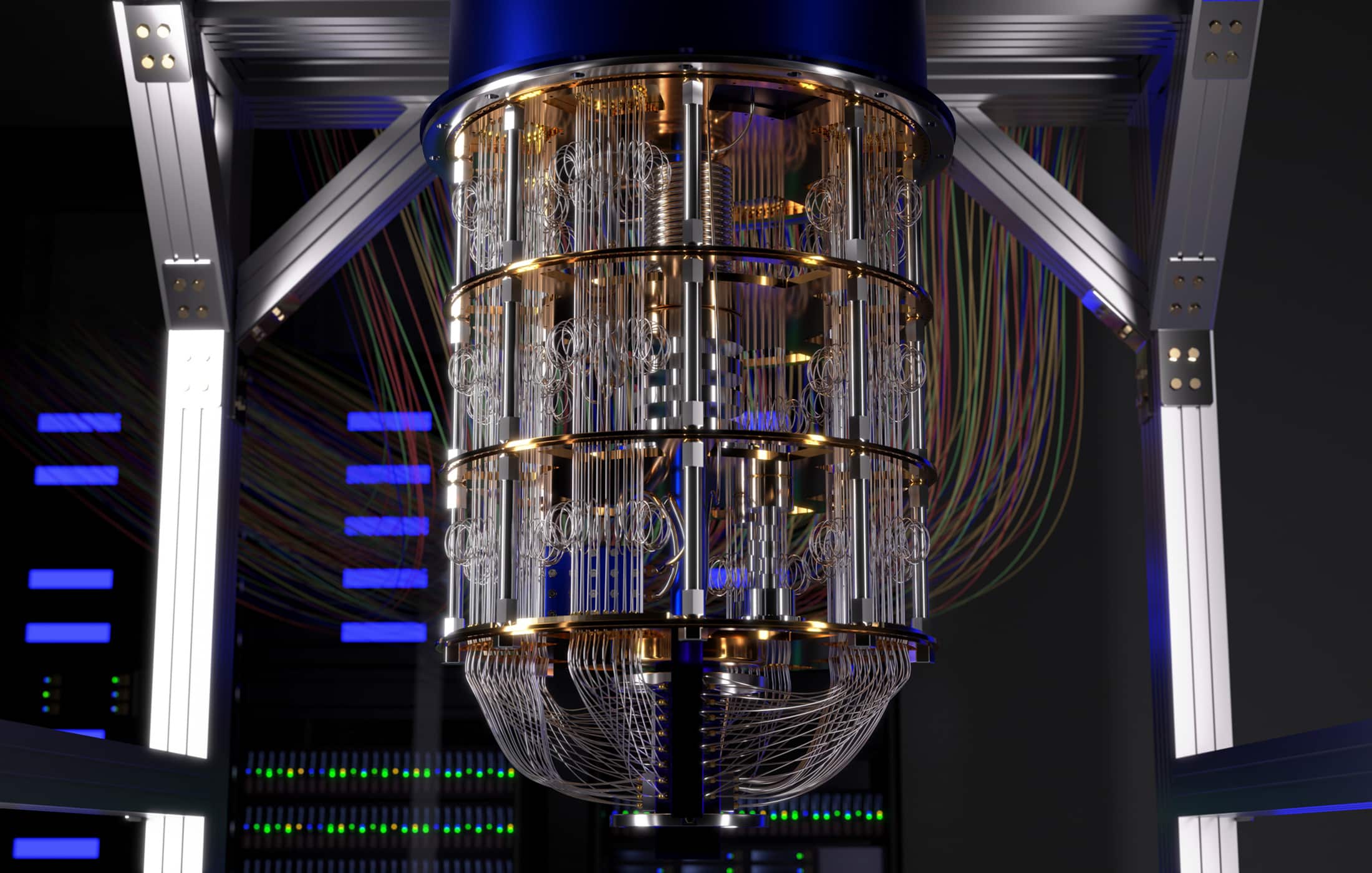
Table of Contents
Transmon qubits are a type of superconducting qubit designed to mitigate charge noise by shunting a Josephson junction with a large capacitor. In other words, a transmon is a superconducting charge qubit that has reduced sensitivity to charge fluctuations. The device consists of a Josephson junction (a nonlinear superconducting element) in parallel with a sizable capacitance, which increases the ratio of Josephson energy to charging energy and thus stabilizes the qubit against charge noise. Transmon qubits are one of the most widely used qubit architectures in quantum computing today, employed in many of the processors built by companies like IBM and Google (as well as startups such as Rigetti Computing).
Key Characteristics
- Josephson Junction Circuit: A transmon is implemented as a superconducting circuit with a Josephson junction (nonlinear inductor) shunted by a large capacitor. This forms an anharmonic oscillator that operates at microwave frequencies (typically in the 3–6 GHz range), which allows quantum states to be manipulated with microwave pulses.
- Reduced Charge Noise Sensitivity: Compared to the earlier Cooper-pair box qubit, transmons are far less sensitive to stray charge on the circuit. The large shunt capacitor makes the qubit’s energy levels nearly independent of background charge fluctuations, significantly reducing dephasing from charge noise.
- Cryogenic Operation: Transmon qubits only function in an ultra-cold environment. They require dilution refrigerator temperatures on the order of ~10–20 millikelvin to maintain superconductivity and quantum coherence. This cryogenic requirement ensures minimal thermal noise but adds complexity to the hardware setup.
How Transmon Qubits Work
A transmon qubit typically consists of a small superconducting island or loop interrupted by a Josephson junction, with a large capacitor shunting the junction. The quantum state of the qubit is encoded in the relative quantum phase across the Josephson junction (which corresponds to the number of Cooper pairs that have tunneled). The junction plus shunt capacitor form a nonlinear resonant circuit with discrete energy levels (qubit levels). By applying microwave-frequency pulses tuned to the energy difference between the qubit’s ground and first excited state, one can drive transitions between these states.
In practice, carefully shaped microwave pulses are used to perform quantum gate operations on transmons, and the qubit state can be read out via a coupled microwave resonator that shifts frequency depending on the qubit’s state. This design allows external control of the qubit while maintaining isolation from most environmental noise.
Advantages of Transmon Qubits
- Improved Coherence Times: Transmons achieve much longer coherence times than earlier charge qubits. State-of-the-art transmon devices can sustain quantum superposition for on the order of 100 microseconds (or more) before losing information, whereas the previous Cooper-pair box designs suffered coherence times only in the few microsecond range. Longer coherence means more operations can be done before errors occur.
- Scalability: The planar fabrication techniques for transmons are compatible with standard silicon chip manufacturing. These qubits are lithographically defined circuit elements, which makes them intrinsically scalable to larger arrays. Tech companies have leveraged this, integrating tens to hundreds of transmon qubits on a single chip (e.g. IBM’s 127-qubit “Eagle” processor is based on the transmon architecture). This scalability is a key reason transmons are a leading approach in quantum computing.
- High-Fidelity Gates: Transmon qubits support fast and high-fidelity quantum gates. With improved calibration and control techniques, single-qubit gate fidelities exceeding 99.9% have been demonstrated on transmons, and two-qubit gate fidelities above 99% are routinely achieved in leading experiments. Such high-fidelity operations are essential for implementing quantum error correction and reliable algorithms.
Challenges:
- Decoherence and Noise: Despite their improved noise resilience, transmon qubits are still susceptible to decoherence. Imperfections in materials (e.g. microscopic two-level defects in device dielectrics), electromagnetic interference, and residual thermal photons can cause energy loss (relaxation) or phase errors in the qubit state. Research continues to push coherence times higher (recent material improvements have extended transmon T<sub>1</sub> relaxation times into the 0.1–0.3 millisecond range), but maintaining quantum states for long durations remains a challenge.
- Cryogenic Requirements: The need for millikelvin temperatures adds significant overhead in any transmon-based quantum computer. Dilution refrigerator systems are expensive and power-intensive, and they limit how easily one can scale up the system’s size. Engineering solutions are needed to manage heat load, wiring, and components inside the cryostat.
- Limited Connectivity and Crosstalk: In a multi-qubit processor, each transmon typically only directly couples to a few neighboring qubits. Designing coupling networks on chip while avoiding unwanted interactions is non-trivial. Increasing the number of qubits can introduce cross-talk (qubits unintentionally influencing each other) and frequency crowding (difficulty finding distinct operating frequencies for each qubit). This limited connectivity means complex multi-qubit operations may require many steps, and calibration to mitigate cross-couplings is laborious. Ensuring that adding more qubits doesn’t dramatically reduce gate fidelity is an ongoing engineering challenge.
Significance in Quantum Computing
Transmon qubits have become foundational to modern superconducting quantum processors. Many breakthroughs in quantum computing have been achieved on transmon-based systems. For example, Google’s landmark quantum supremacy experiment in 2019 used a 53-qubit transmon processor called “Sycamore” to perform a computation infeasible for classical supercomputers (a random circuit sampling task). IBM has also built its quantum computing roadmap around transmon qubits, steadily increasing qubit counts and improving performance metrics. IBM’s processors have demonstrated increasing Quantum Volume (a holistic measure of quantum computing power); for instance, IBM doubled the quantum volume of its best system to 256 in 2022 through improvements in transmon qubit coherence and gate fidelity. The relative ease of fabrication, control, and integration of transmons has made them a leading platform as the field progresses toward larger, more reliable quantum computers. In the near term, transmon-based NISQ (noisy intermediate-scale quantum) devices are enabling experiments in quantum algorithms and error correction, and in the long term they are a strong candidate for realizing fault-tolerant quantum computing architectures.
Impact on Cybersecurity
- Cryptographic Threats: As transmon-based quantum processors continue to improve, they move closer to the scale needed to run Shor’s algorithm and other cryptographically relevant quantum algorithms. A sufficiently powerful quantum computer could break widely used public-key cryptosystems (like RSA and elliptic-curve encryption) by factoring large numbers or computing discrete logarithms efficiently. The prospect of such capabilities in the future poses a serious threat to current encryption methods, driving concern in the cybersecurity community.
- Post-Quantum Security Research: The advancement of superconducting qubits like the transmon is directly influencing the push for post-quantum cryptography. Anticipating eventual quantum attacks, researchers and standards bodies are developing quantum-resistant encryption algorithms and protocols. Transmon qubits also provide a testbed for quantum-safe technologies; for example, small-scale quantum processors can experimentally verify algorithms for cryptography that are designed to be safe against quantum adversaries, and can interface with quantum communication systems for protocols like quantum key distribution (QKD). In short, the rise of transmon-based quantum computers is catalyzing efforts to secure data in a future where quantum attacks are possible.
Summary
Transmon qubits are one of the most successful and widely adopted qubit designs in superconducting quantum computing. They combine enhanced noise resilience (thanks to the shunting capacitor design), relatively long coherence times, and compatibility with microfabrication techniques, which together have accelerated progress in the field. High-fidelity control of transmons has enabled experimental demonstrations of complex algorithms and paved the way toward scalable quantum computing. However, challenges such as decoherence and the necessity of cryogenics mean continued engineering refinements are required. Overall, transmon-based systems are at the forefront of today’s quantum computing breakthroughs, and their development carries significant implications for cryptography and cybersecurity in the coming quantum era.