Zuchongzhi 1.0: China’s New Superconducting Processor
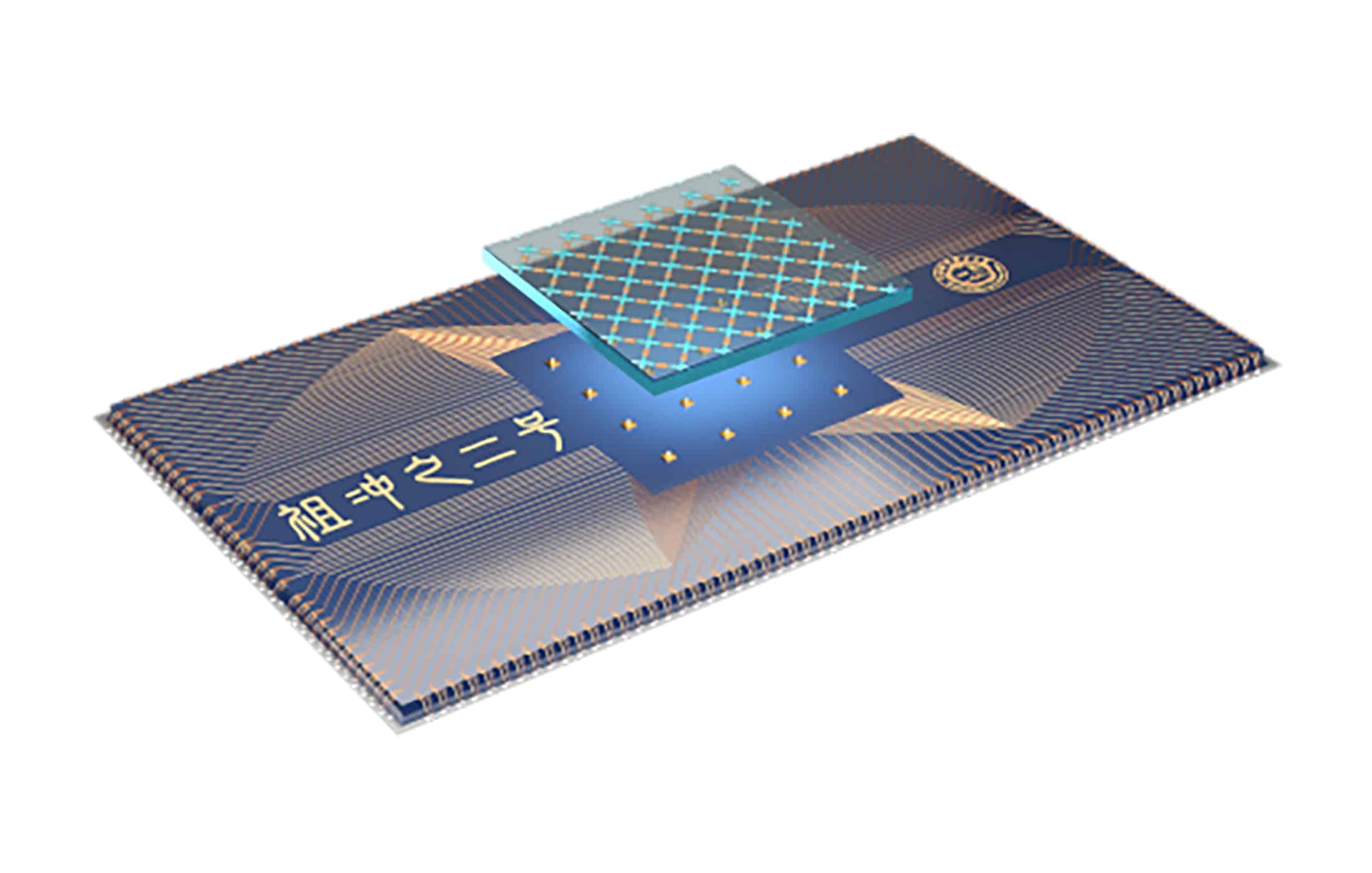
Table of Contents
In May 2021, scientists at the Chinese Academy of Sciences (CAS) unveiled Zuchongzhi 1.0, a 62-qubit programmable superconducting quantum computer that set a new benchmark in the quantum computing race. Named after a 5th-century Chinese mathematician, Zuchongzhi 1.0 contains the largest number of superconducting qubits ever assembled in a single processor so far. This breakthrough machine, announced by the CAS Center for Excellence in Quantum Information and Quantum Physics and published in Science in May 2021, achieved a milestone experiment: two-dimensional programmable quantum walks using all 62 qubits. The achievement underscored China’s growing role in cutting-edge quantum computing and hinted at a new era of quantum advantage on a programmable platform.
A Programmable 62-Qubit Quantum Computer Sets a New Record
Zuchongzhi 1.0 is a 62-qubit superconducting processor, built on an 8×8 two-dimensional grid of transmon qubits (with a few sites unoccupied). Each qubit is a tiny superconducting circuit that can hold a quantum bit of information, and neighboring qubits are linked by tunable couplers that allow controlled interactions. In their first demonstration, the research team used this device to perform high-fidelity single- and two-particle quantum walks – essentially letting one or two quantum “walkers” hop around the grid in a superposition of paths. It’s akin to particles taking random walks on an 8×8 chessboard, exploring many routes at once thanks to quantum superposition. By programming different patterns of couplings, the team implemented a kind of Mach-Zehnder interferometer on the chip, where the wandering particles split and recombine along different pathways. The resulting interference fringes, observed over many runs, verified that the qubits were entangled and coherently controlled across the 62-node lattice. Achieving such two-dimensional programmable quantum walks is a major milestone – it demonstrates that the processor can handle complex quantum evolutions in a highly controllable way, an essential step toward general-purpose quantum computing.
This feat also marked a world record in superconducting qubit count. At 62 qubits, Zuchongzhi 1.0 edged past other superconducting processors of the day. Google’s famous Sycamore chip, for instance, had 53 qubits, and IBM’s largest operational device at the time contained 65 qubits. As CAS highlighted, the Zuchongzhi processor boasted “the largest number of superconducting qubits so far in the world,” indicating China had reached parity with – and in some aspects, surpassed – the U.S. efforts in quantum hardware scale. “The 62-qubit Zu Chongzhi processor at least showed that China is at the same level as its US counterparts in the field of superconducting quantum computing,” noted Yuan Lanfeng, a USTC researcher, emphasizing the significance of this achievement. Beyond sheer qubit count, Zuchongzhi 1.0’s programmability and high fidelity operations signaled that it wasn’t just big, but also functional – a key consideration as quantum machines grow. The successful quantum walk experiments suggested that the processor could execute arbitrary quantum algorithms on dozens of qubits, reinforcing that “such a device can be applied to universal quantum computing” rather than a one-off trick.
Quantum Advantage: From Google’s Sycamore to Zuchongzhi
The unveiling of Zuchongzhi 1.0 came on the heels of a series of quantum computing “firsts” that defined the field’s global momentum. In October 2019, Google grabbed world headlines by announcing it had achieved quantum supremacy with its 53-qubit Sycamore processor. Sycamore performed a carefully chosen task – random quantum circuit sampling – in about 200 seconds, a task the Google team estimated would take a classical supercomputer thousands of years. This demonstration was the first to experimentally show a quantum device outperforming any classical computer on a specific problem, an inflection point termed quantum computational advantage (or formerly “supremacy”). IBM, another leader in superconducting qubits, contested Google’s claim by devising improved simulation algorithms on classical supercomputers, arguing the task could be done in a matter of days under certain conditions. Nonetheless, Google’s experiment stood as a landmark proof-of-concept: quantum machines had crossed a threshold in computational power.
China quickly answered with its own approach to quantum advantage, but via a different technology. In December 2020, Jiuzhang 1.0, a photonic quantum computer developed by Pan Jianwei’s team (the same group behind Zuchongzhi), demonstrated quantum advantage using 76 detected photons in a specialized task called Gaussian boson sampling. That experiment, published in Science, was the second time ever that a quantum device beat classical computers at a task, after Google’s Sycamore. However, Jiuzhang’s optical approach – though blazingly fast at one specific calculation – is not a general-purpose, programmable computer. It solves one problem (sampling the output of a random optical interferometer) and cannot be easily reprogrammed for other algorithms. Zuchongzhi 1.0, by contrast, is a fully programmable gate-based quantum computer, meaning it can in principle run any quantum circuit or algorithm (within the limits of its size and coherence) just like Google’s and IBM’s superconducting chips. This made Zuchongzhi 1.0 the first platform outside of Google’s to aim for quantum advantage with a universal programmable quantum processor.
Indeed, one of the goals for Zuchongzhi 1.0 was to perform the random circuit sampling (RCS) task that Sycamore had tackled, but with more qubits and greater complexity. The team held off on immediately claiming quantum advantage in the initial Science publication, focusing on the quantum walk demonstration as a showcase of the machine’s capability. But behind the scenes, they were already gearing up to push RCS to new heights. Random circuit sampling involves executing a sequence of random gate operations on many qubits and sampling the output bitstrings; it’s designed to be hard to simulate classically, thus serving as a yardstick for quantum power. With 62 qubits at hand (and shortly after, a slight upgrade to 66 qubits), the USTC team expected to outrun classical simulation by a comfortable margin.
They soon proved it. By mid-2021, Pan’s group reported that Zuchongzhi could indeed generate quantum random circuit samples far beyond the reach of classical algorithms. Using a subset of 56 qubits out of the chip and running up to 20 layers of random gates, they estimated the computation would take an impractical amount of time on any existing supercomputer. In technical terms, the 56-qubit, 20-cycle random circuit sampled from Zuchongzhi 1.0 (and its slightly refined successor) would require orders of magnitude more computing resources to simulate than Google’s 53-qubit circuit. The team concluded that their system “outperformed Google’s 53-qubit processor, Sycamore”, firmly re-establishing a quantum advantage as of 2021. In other words, if Google opened the door to quantum advantage, Zuchongzhi barged through it with even more qubits – making the computational gap between quantum and classical even wider. Barry Sanders, a quantum computing expert, noted that these rapid advances in sampling experiments have made it “increasingly unlikely that there could be efficient classical algorithmic alternatives” to spoof the quantum results.
Meanwhile, IBM’s approach to quantum computing in this era provided a counterpoint. Rather than chasing immediate supremacy demonstrations, IBM focused on steadily scaling up devices while maintaining reliability. In 2020, IBM had prototyped a 65-qubit superconducting chip (codenamed Hummingbird) and introduced the concept of quantum volume to measure a quantum computer’s all-around capability (not just qubit count). IBM also laid out an ambitious roadmap towards 127-qubit, 433-qubit, and eventually 1000+ qubit processors in the coming years. By November 2021, IBM did unveil “Eagle,” a 127-qubit chip, but that came after Zuchongzhi’s debut and did not yet run quantum advantage experiments. In the context of early 2021, IBM’s 65-qubit processor had comparable scale to Zuchongzhi, but it hadn’t been pushed to perform a task that could challenge classical computing. Thus, Zuchongzhi 1.0’s success put China on the short list of teams achieving a quantum advantage experiment, alongside Google’s and Pan’s own photonic system. It showcased an alternative design and engineering prowess that matched the best of the West, intensifying the global competition in quantum computing.
Technical Innovations Under the Hood
Building Zuchongzhi 1.0 was not just about adding more qubits – it required significant engineering innovations to preserve quantum coherence and control across the large array. Like Google and IBM’s devices, Zuchongzhi’s qubits are transmons (superconducting circuits that behave like artificial atoms) chilled to millikelvin temperatures. The team adopted a two-chip architecture: one chip holds the qubits and coupler circuits, and another chip sits above it containing control wiring, with tiny indium bump bonds connecting the layers. This 3D packaging (often called flip-chip integration) minimizes the footprint and allows dense connectivity without too many cross-overs, an important technique for scaling up qubit count. In Zuchongzhi’s design, each qubit is connected to its neighbors via a tunable coupler – essentially an extra qubit junction that can be electrically tuned to turn the interaction on or off. When the couplers are “off,” each qubit is largely isolated and can undergo single-qubit rotations without disturbing others; when “on,” a pair of qubits can perform an entangling two-qubit gate. This tunable coupling strategy was key to achieving parallel operations across the chip and reducing crosstalk, thus maintaining high fidelity even as the number of qubits grew.
The performance metrics of Zuchongzhi 1.0 reflect these careful design choices. In their experiments, the team reported single-qubit gate fidelities on the order of 99.9% and two-qubit gate fidelities around 99.5%, on par with the best reported by Google and IBM.
Another notable aspect is how Zuchongzhi 1.0 balanced the quantity vs. quality trade-off. Simply scaling up qubit count is futile if errors scale up too. The USTC team’s approach was to increment qubit count in a manageable way (from a smaller 2019 prototype up to 62) while keeping error rates low through improvements in materials, fabrication, and control software. The result is a machine that not only has a lot of qubits on paper but can actually entangle dozens of them in practice for a computation. “The core objective is to synchronously increase the number of integrated qubits and improve their performance,” noted the CAS report, aiming ultimately to “achieve exponential acceleration in processing speed of specific problems.” Zuchongzhi 1.0’s ability to carry out a two-dimensional quantum walk – which requires sustained coherence across many sequential gate operations – is strong evidence that those objectives are being met. In the longer run, techniques tested in Zuchongzhi (like tunable couplers and 2D arrangements) will be vital for implementing error-correcting codes on superconducting qubits. In fact, shortly after the 62-qubit milestone, the same team went on to demonstrate a small surface code (a type of quantum error correction) on a 17-qubit subset, showing they are already laying the groundwork for fault-tolerant architectures.
Broader Implications and Outlook
Zuchongzhi 1.0’s debut carries implications beyond the laboratory experiment it performed. For one, it cemented China’s position as a leading player in quantum computing, being the only country to achieve quantum computational advantage in two different physical platforms (photons and superconductors) as of 2021. This scientific milestone has fed into a broader technology race: quantum computing is now seen as a strategic frontier, much like space or nuclear technology during the Cold War. Governments and tech companies around the world are pouring resources into quantum R&D, and breakthroughs like Zuchongzhi add urgency. The CAS team themselves acknowledged that the development of quantum computers has “become the focus of competition among countries around the world.” Every increase in qubit count and computational power not only pushes the science forward but also raises the stakes in terms of national investment and talent development in this field.
In industry, the near-term practical impact of Zuchongzhi 1.0 is subtle but significant. The specific task it tackled – random circuit sampling or quantum walks – doesn’t have direct commercial application; these are benchmark problems chosen for their computational hardness. However, the techniques and technologies proven by Zuchongzhi can translate to more useful algorithms down the road. For example, quantum sampling methods might be repurposed for simulating quantum chemistry or solving optimization problems, which have real-world value in drug discovery, materials science, and logistics. There is optimism in the community that NISQ-era processors (Noisy Intermediate-Scale Quantum) could be tuned to address certain practical problems faster than classical computers, though this has yet to be clearly demonstrated. Zuchongzhi 1.0 and its successors provide a platform on which such exploratory algorithms can run with an unprecedented number of qubits. Each improvement – a few more qubits here, a bit less error there – expands the range of feasible quantum computations for researchers to experiment with.
On the flip side, rapid progress in quantum computing also keeps the spotlight on cybersecurity concerns. A fully error-corrected quantum computer of sufficient size could one day run Shor’s algorithm to break RSA encryption, for instance, undermining current cryptographic protocols. While Zuchongzhi 1.0 is still far from that level (it does not implement Shor’s algorithm and has no error correction yet), every quantum milestone reminds us that what’s academically cutting-edge today could lead to practical capabilities tomorrow. It’s no coincidence that efforts in post-quantum cryptography (developing quantum-resistant encryption) have accelerated in parallel with advances like Sycamore and Zuchongzhi. Quantum computers “hold the promise of exponentially accelerating” the solving of certain problems in cryptography and beyond, which is both exciting and challenging for society. The consensus among experts is that we are still many years away from a quantum computer that threatens public-key encryption, but the trajectory is clear – and Zuchongzhi 1.0 is an important data point on that trajectory.