Quantum Use Cases in Healthcare & Medical Research
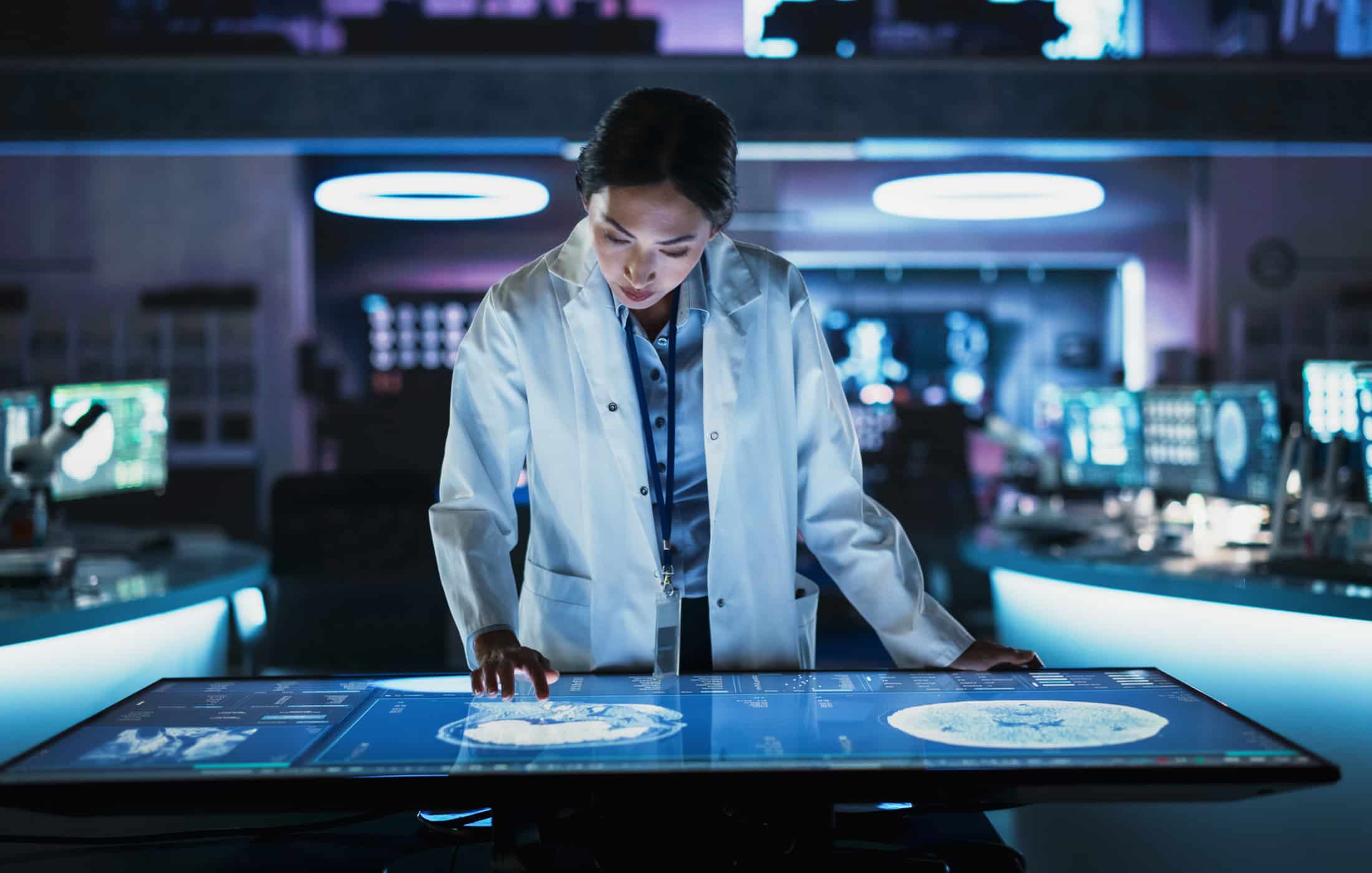
Table of Contents
Introduction
Quantum computing is an emerging technology that leverages principles of quantum mechanics to perform calculations far beyond the reach of classical computers. By exploiting phenomena like superposition and entanglement, quantum computers (QCs) can process vast combinations of data simultaneously, offering the potential to solve complex biomedical problems with unprecedented speed and efficiency. This matters for healthcare because many challenges in medicine – from simulating intricate molecular interactions to analyzing enormous patient datasets – are computationally intensive or even intractable on today’s machines. With quantum computing’s hitherto unattainable capabilities, researchers envision transformative benefits: for example, handling exponentially growing health data or accelerating tasks like drug discovery and genome analysis that currently take months. Indeed, during the COVID-19 pandemic, the slow pace of genomic sequencing for emerging viral variants underscored the need for new computing approaches; quantum techniques promise a way to speed up such critical analyses in future public health crises. In short, quantum computing’s ability to tackle complexity at scale gives it revolutionary potential in healthcare – from earlier disease detection to highly personalized treatments – even if its full impact is only beginning to be explored.
Current Developments
Around the world, academia, industry, and government are increasingly investing in quantum computing for healthcare. A landmark example came in 2023, when IBM and Cleveland Clinic unveiled the first quantum computer dedicated solely to biomedical research. Housed on Cleveland Clinic’s campus, this IBM Quantum System One is part of a 10-year Discovery Accelerator partnership aimed at dramatically speeding up discovery of new medicines and treatments. Pharmaceutical companies are likewise exploring quantum algorithms: Biogen has collaborated with Accenture to expedite the search for neurological disease therapies using quantum computing, and Moderna partnered with IBM to apply QCs in mRNA vaccine research. Public and private collaborations are proliferating – for instance, New York University’s Langone Health is working with the U.S. Department of Energy’s Fermilab to apply quantum computing in MRI analysis, marking the first healthcare-focused partnership for Fermilab’s quantum research center. Governments, too, are fostering innovation: the U.S. National Institutes of Health launched a Quantum Biomedical Tech (Qu-BIT) program in 2024 to advance quantum sensing and computing for translational medicine, and the U.K. recently funded a £24 million Quantum Biomedical Sensing Hub – the first British quantum research hub dedicated to healthcare – to develop quantum-based early diagnostics involving leading universities, the National Health Service, and industry. These developments signal a growing momentum. Although today’s quantum hardware is still rudimentary, major healthcare players are positioning themselves through research initiatives and strategic investments so that they can capitalize on quantum breakthroughs as they arise.
Industry-Specific Use Cases
Medical Imaging & Diagnostics
Quantum technologies are poised to enhance medical imaging both in how images are obtained and how they are analyzed. On the hardware side, quantum-enhanced imaging techniques promise sharper and faster scans. For example, researchers are developing quantum MRI systems that exploit quantum coherence and entanglement to greatly improve sensitivity. Traditional MRI relies on relatively weak signals from nuclear spins, but quantum sensors can detect minute magnetic field changes with far greater precision, enabling higher-resolution images of soft tissues and even neural activity. The result could be earlier and more accurate detection of abnormalities like tumors, with shorter scan times and less discomfort for patients. Such quantum-based imaging advancements, though still experimental, hint at a future of non-invasive diagnostics that can reveal disease markers at much smaller scales or sooner in the disease process than current imaging modalities.
Beyond image acquisition, quantum computing can supercharge how we interpret diagnostic data. Quantum algorithms – including quantum neural networks and support vector machines – can process complex medical datasets and identify subtle patterns that classical AI might miss. This capability is especially useful in radiology and pathology, where pattern recognition is key. For instance, QCs could sift through thousands of MRI or CT scans in parallel to flag the faint early signs of neurodegenerative disease, or to detect tiny tumors that elude human eyes. Google’s Quantum AI team and D-Wave have already been researching quantum machine learning for cancer diagnostics, showing that quantum processors can handle massive imaging and genomic datasets to improve early cancer detection. In one approach, a quantum annealer evaluates many possible image segmentation solutions simultaneously – essentially tunneling through an enormous solution space – which can significantly speed up identification of suspicious lesions. Although these are still prototype efforts, they demonstrate the potential for quantum-enhanced diagnostics that combine advanced imaging with AI. In the coming years, we may see quantum computing routinely aiding radiologists and clinicians, leading to faster and more accurate diagnoses from medical images and other diagnostic tests.
Hospital & Healthcare Systems Optimization
Modern hospitals are extraordinarily complex operations – coordinating staff schedules, operating rooms, bed assignments, supply chains, and more – and even small inefficiencies can impact patient care. Quantum computing offers new ways to optimize these logistics problems that strain classical systems. One promising approach is using quantum optimization algorithms (or quantum-inspired algorithms) to evaluate millions of scheduling and resource allocation possibilities virtually instantaneously. For example, quantum computers could help generate optimal nurse and physician shift schedules that ensure adequate coverage at all times, minimizing both understaffing and overtime. Early simulations have shown dramatic improvements: one study using quantum-inspired methods reduced patient wait times by up to 40% by better managing patient flow and bed assignments. Another experiment applied a quantum-inspired optimizer to ambulance dispatch routing, cutting emergency response times by an estimated 20%. These gains come from the ability of quantum algorithms to handle the combinatorial explosion of possibilities in large logistics problems, exploring many what-if scenarios in parallel rather than by trial-and-error.
Optimizing hospital operations with quantum tools could translate into tangible benefits: shorter ER wait times, fewer delayed procedures, and more efficient use of limited resources like ICU beds. It also has financial upside – studies have projected that quantum-driven resource allocation could trim hospital operational costs by around 15% through better inventory management and scheduling. While quantum computers powerful enough to fully optimize an entire hospital in real-time are still on the horizon, even today’s early quantum processors (and hybrid algorithms running on classical hardware) are being tested on these problems. Companies and research labs are collaborating with hospitals on pilot projects for scheduling, and startups are emerging with “quantum-inspired” software for healthcare logistics. Over the next few years, as quantum hardware improves, we can expect healthcare systems optimization to be one of the first areas where quantum computing delivers practical value, helping administrators make data-driven decisions to streamline care delivery and ultimately improving patient outcomes and access.
Personalized Treatment Plans
One of the most exciting prospects of quantum computing in medicine is the ability to truly personalize treatment plans by analyzing a patient’s data in richer detail than ever before. In precision medicine today, doctors consider factors like genetics, lab results, and medical history to choose therapies – but often this analysis is limited by computational tools. Quantum-enhanced AI could change that. Quantum algorithms might digest a patient’s entire genome, proteome, lifestyle factors, and clinical records as one enormous dataset, searching for patterns that indicate which treatment would work best. For example, a quantum computer could rapidly scan through billions of genetic and molecular data points to identify which drug a tumor is most likely to respond to, tailoring cancer therapy to that individual’s unique tumor profile. Researchers have noted that Grover’s quantum search algorithm could comb vast genomic databases dramatically faster than classical methods, potentially uncovering subtle gene variants that influence treatment response. In essence, quantum computing might enable “hyper-personalized” care by factoring in far more variables about a patient than traditional computing can manage.
We are already seeing early steps toward this vision. Quantum computers have been used in prototypes to optimize radiation therapy plans for cancer patients: by evaluating countless beam angles and dose distributions, a QC can compute an optimal plan that maximizes tumor kill while sparing healthy tissue, all within feasible computation time. This means radiation oncologists could one day generate highly precise, patient-specific treatment plans in minutes, something that currently takes many hours of supercomputing. More broadly, quantum algorithms can integrate genomic and clinical data to predict how a particular patient will respond to a list of possible therapies. In trials, this has been demonstrated for tailoring chemotherapy based on genetic markers – essentially using quantum-powered analytics to pick the drug (or drug combination) with the highest probability of success for that individual. The convergence of quantum computing and AI thus holds promise to become the ultimate clinical decision support tool: a doctor might input a patient’s profile and, with quantum enhancements, receive real-time guidance on the best treatment strategy, even for complex cases with multiple comorbidities. Although significant validation and regulatory approval will be needed, the future where treatment plans are optimized not just from general evidence but from quantum-crunched insights into each patient’s data is drawing closer.
Medical Robotics & Surgical Planning
In surgical care, precision and planning can make the difference between a routine operation and a risky one. Quantum computing could augment medical robotics and surgical planning by handling the staggering complexity of human physiology in simulations. One near-term impact may be in pre-operative planning: before a complicated surgery, surgeons often run simulations or rehearse using patient imaging data. Quantum computers can take this to the next level by simulating intricate biomechanical interactions in the body with far greater accuracy. For instance, in orthopedic surgery planning, a QC could model how a specific patient’s bones, muscles, and a proposed implant will interact under various movements – something classical computers struggle to do in real time. Researchers have speculated that quantum-enhanced simulation could provide surgeons with valuable previews of surgical outcomes in a virtual environment. A surgeon might “test” different surgical approaches on a quantum-powered digital twin of the patient, seeing which technique yields the best stability or fastest healing, and then go into the operating room with a data-backed plan tailored to that individual. This kind of quantum-augmented planning could improve surgical precision and reduce intraoperative surprises.
Looking further ahead, the advent of fault-tolerant quantum computers may enable advances in robotic-assisted surgery itself. Robotic surgical systems today (like the da Vinci robot) rely on classical algorithms for things like motion control, vision, and instrument navigation. In the future, quantum optimization algorithms could refine the control of these robots – for example, calculating an optimal path for a surgical instrument around delicate structures in milliseconds, or adjusting on the fly to dynamic changes in tissue resistance. Experts predict that quantum computing will accelerate the development of next-generation surgical tools, perhaps allowing real-time integration of massive data (like live MRI or ultrasound feedback) into robotic control. We could see “smart” surgical robots that use quantum processors to instantaneously analyze sensor data and guide instruments with uncanny precision, enabling surgeries that are less invasive and more accurate than ever. Moreover, quantum-enhanced diagnostics (as mentioned earlier) could feed into surgery – e.g., an AI that flags microscopic tumor remnants using quantum analysis could guide a robot to excise them during an operation. While these scenarios remain speculative, the convergence of quantum computing and surgical robotics holds tremendous promise. Future complex procedures – from neurosurgeries to organ transplants – might be executed by teams of surgeons and quantum-driven robots working in tandem, significantly improving patient safety and surgical outcomes.
Epidemiology & Public Health
Public health and epidemiology involve massively complex systems – entire populations, global travel networks, multifactorial disease dynamics – which are challenging to model and predict. Quantum computing offers tools to handle this complexity for better outbreak prediction and health policy planning. One application is in epidemic surveillance and modeling. Quantum computers can analyze massive datasets from disease surveillance (symptom reports, mobility data, social media signals, etc.) in near real-time, spotting patterns that might herald a disease outbreak much faster than classical systems. For example, a quantum machine learning model could continuously ingest streams of public health data and identify subtle anomalies – a spike in ER visits or unusual symptom clusters – that might indicate an emerging infectious disease, raising an early alarm days or weeks sooner. Quantum processing also shines in running complex simulations. It could enable far more detailed epidemiological models that factor in many variables (human behavior, climate, virus mutations, etc.) simultaneously. Researchers suggest this could improve our understanding of how viruses evolve and spread; a quantum simulation might accurately depict how an avian flu virus mutates and jumps to humans, leading to better preventive strategies to stop it. In effect, quantum computing could give public health officials a “big picture” model that is both faster and more predictive, akin to how advanced weather models improve storm forecasts.
Quantum technology can also assist in pandemic response planning by solving optimization problems related to interventions. During COVID-19, policymakers struggled to design lockdowns or vaccine distributions that balance health benefits with economic and social costs. Quantum algorithms are being tested on such problems – one study used a hybrid quantum model to determine optimal lockdown schedules across cities and found it could reduce COVID cases while maintaining economic activity better than classical approaches. In practice, this might mean a quantum computer evaluating countless combinations of intervention policies (timing of lockdowns, travel restrictions, resource deployment) to find the strategy that best controls an outbreak with minimal disruption. Likewise, distributing limited medical resources in a pandemic (like ventilators or vaccines) is an NP-hard optimization task that quantum solvers could tackle, potentially outputting distribution plans that save more lives. Additionally, health policy planning could benefit: quantum models could help policymakers test long-term scenarios – for example, how different vaccination programs or preventive screenings would impact population health over decades – by quickly crunching through complex demographic and biomedical data. Of course, using quantum outputs in public health will require trust and validation, but the ability to derive insights from unimaginable volumes of data could greatly enhance how we manage public health threats. In the future, “quantum epidemiology” might guide officials in containing outbreaks, optimizing public health interventions, and crafting evidence-based policies underpinned by quantum-processed evidence.
Data Security & Medical Records
As healthcare goes digital, securing sensitive medical data is paramount – and here quantum technology is both a threat and a solution. On one hand, powerful quantum computers pose a serious data security risk: they could eventually crack the encryption algorithms (like RSA and ECC) that currently protect electronic health records, medical devices, and telemedicine communications. This looming event, often dubbed “Q-Day,” implies that any encrypted health information stolen today could be decrypted in the future once large-scale quantum computers are available. Patient records are especially vulnerable because they remain valuable for a lifetime – for example, a hacker might steal encrypted medical archives now and years later use a quantum attack to reveal private diagnoses or genomic data. This has sparked urgency in developing quantum-resistant encryption for healthcare. Efforts are underway (led by bodies like NIST) to deploy new cryptographic algorithms that even quantum computers can’t easily break. At the same time, quantum physics offers tools to enhance security. Quantum cryptography, particularly Quantum Key Distribution (QKD), allows sharing encryption keys in a manner that is theoretically impervious to eavesdropping – any interception of the key is immediately detectable due to the laws of quantum mechanics. Hospitals and research institutions have started experimenting with QKD for securing sensitive data transmissions (for instance, between hospital sites), ensuring that medical records or imaging scans sent over networks cannot be intercepted without alerting the parties. Additionally, post-quantum cryptography (new algorithms run on classical computers but designed to resist quantum attacks) is being integrated into healthcare data standards as a preventive measure. In telemedicine, where doctor-patient consultations and vital signs travel over the internet, quantum-secure communication channels may soon be adopted to maintain confidentiality in the face of quantum hacking threats. In summary, the healthcare sector is racing to both upgrade its defenses and leverage quantum technology for security. The goal is that by the time universal quantum computers arrive, health data will be secured by quantum-proof or quantum-powered encryption – turning the quantum threat into an opportunity for even stronger privacy protections than we have today.
The Arrival of Universal Quantum Computing
When fully fault-tolerant quantum computers become a reality – machines with millions of stable qubits capable of error-corrected, universal computation – the impact on healthcare and medical research will be profound. These future quantum computers are expected to solve problems that are effectively impossible for any classical supercomputer, heralding an era of computational abundance for medicine. On the beneficial side, we could see astonishing capabilities: near-instant “supersonic” drug design and virtual drug testing, AI-driven in silico clinical trials on realistic virtual patients, real-time whole-genome analysis for anyone who needs it, and predictive models that can simulate entire organ systems or epidemics in exquisite detail. Researchers and futurists imagine scenarios like running a full clinical trial virtually – a quantum computer could model thousands of virtual humans with various genetics and conditions to test a new therapy in minutes, potentially identifying efficacy and side effects before a real human trial begins. Discovering new cures might accelerate dramatically as QCs churn through chemical libraries and protein targets at blazing speeds, finding viable drug candidates in days rather than years. Even day-to-day healthcare could be transformed: diagnostics may become so advanced that diseases are caught years earlier through subtle markers in one’s data, and treatment planning might account for complexities (like drug interactions, co-occurring diseases, lifestyle factors) in a way that truly “personalizes” medicine at scale. In short, a fault-tolerant quantum computer would act as an unparalleled engine of medical insight, helping us decode biological puzzles and design interventions with a speed and precision that today seem unimaginable.
However, the arrival of universal QCs will also be disruptive. There is genuine concern about a “Q-Day” scenario on the cyber front: once quantum machines can easily break current encryption, hospitals and health systems worldwide will face a scramble to re-encrypt or risk massive data breaches. This necessitates an ecosystem-wide upgrade to post-quantum cryptography (already underway, but not yet fully deployed) to protect patient privacy and medical intellectual property. Beyond security, fault-tolerant QCs could render many existing clinical software tools and even some medical training outdated. For example, algorithms doctors rely on (for imaging, genomics, prognosis) might be quickly outclassed by quantum-driven versions, pressuring healthcare providers to adapt to new decision-support systems that they may not fully understand internally. There’s also the risk of inequity and disruption if access to quantum computing is uneven – leading institutions with early access will leap ahead in research and care capabilities, potentially widening gaps between well-funded health systems and those without quantum resources. Regulators and medical institutions will need to manage the transition carefully: validating quantum-derived medical insights for safety and efficacy, and integrating them in a way that augments rather than confuses clinical workflows. In essence, universal quantum computing will be a double-edged sword for healthcare. It promises extraordinary breakthroughs, but it will challenge us to upgrade our infrastructure, retrain our workforce, and rethink policies at an unprecedented pace. The good news is that leaders are aware of these coming shocks: the healthcare industry is already planning for quantum-induced disruptions (like the encryption issue) and strategizing on how to harness the benefits while minimizing any harmful effects. If we can navigate the transition, the long-term outcome could be overwhelmingly positive – a healthcare system supercharged by quantum computing, delivering cures and insights that were once considered science fiction.
Sector Preparation & Responses
Healthcare institutions, research centers, and technology providers are not waiting idly for quantum computing to arrive – many are actively preparing for this paradigm shift. A top priority is developing a quantum-ready workforce. Universities and hospitals have begun rolling out specialized training programs to cultivate professionals fluent in both quantum tech and life sciences. For example, in January 2025 Miami University and Cleveland Clinic announced a partnership to establish Ohio’s first degree programs and research experiences in quantum computing, with the explicit aim of creating talent to leverage quantum computing in healthcare. This kind of initiative will prepare engineers and clinicians who understand quantum algorithms and can apply them to biomedical problems. More broadly, there’s a push for “quantum literacy” across the health sector. Experts argue that clinicians, healthcare data scientists, and IT staff need at least a baseline understanding of quantum principles so they can judiciously adopt quantum tools when the time comes. To that end, workshops and courses on quantum computing for healthcare are popping up at conferences, and some medical schools have begun including discussions of AI and quantum futures in their curriculum. The goal is to avoid a knowledge gap; when quantum-powered software or devices start appearing in hospitals, staff should be ready to evaluate and use them, rather than be intimidated by black-box magic.
Healthcare organizations are also forming strategic collaborations and testbeds to get hands-on experience with quantum technology. Many leading hospital systems (Cleveland Clinic among them) have joined networks with quantum hardware providers like IBM, D-Wave, and Google to run pilot projects and share knowledge. On the regulatory and policy front, preliminary steps are being taken to update frameworks for quantum medical technology. Regulators in the U.S. and EU are considering how to evaluate quantum-powered medical devices and algorithms, recognizing that these might not fit neatly into existing approval processes. Proposals include creating regulatory sandboxes where quantum health tech can be tested under oversight, and developing new validation protocols tailored to quantum AI (for example, ensuring a quantum-derived diagnostic algorithm’s outputs are explainable and reproducible). International standards bodies are also beginning to discuss interoperability and safety standards for quantum health applications, aiming to avoid a patchwork of rules. Meanwhile, governments are funding public-private consortia to accelerate progress: the NIH’s Qu-BIT program (noted earlier) not only funds research but also convenes interdisciplinary teams to brainstorm quantum solutions for biomedical challenges. Similarly, the EU’s and UK’s quantum initiatives are including healthcare use cases in their roadmaps, ensuring that healthcare voices are at the table as national quantum strategies unfold. Major tech companies are doing their part by offering quantum cloud platforms accessible to researchers – for instance, IBM’s Quantum Network provides free and priority access to certain quantum processors for academic and healthcare research teams. This lets hospitals and biotech startups experiment with quantum algorithms now, identifying practical issues and building expertise. In summary, the sector’s response to the coming quantum revolution is proactive: build knowledge, shape policy, and get involved early. By investing in people (through education) and in partnerships (through collaborations and consortia), healthcare stakeholders are striving to be ready to hit the ground running as quantum computing matures.
Challenges and Risks
Despite its potential, integrating quantum computing into healthcare faces significant challenges and risks that must be addressed. Technical limitations remain the most immediate barrier. Today’s quantum hardware (so-called NISQ – Noisy Intermediate-Scale Quantum devices) have only tens or hundreds of qubits that are extremely prone to errors and decoherence. Performing useful computations on them is difficult, and scaling up to the large, stable quantum computers needed for impactful healthcare applications is an ongoing engineering struggle. Qubits require ultra-cold, isolated conditions to function, and even then errors accumulate rapidly, necessitating error-correction schemes that themselves demand many physical qubits. This means that in the near term, tasks like accurately simulating a complex protein or running a full patient-data analysis on a quantum computer may be out of reach. Additionally, programming quantum computers and formulating healthcare problems in quantum terms is non-trivial – there is a steep learning curve and a lack of user-friendly software, which slows adoption. In essence, the technology is not yet “plug-and-play.” It could be years or decades before truly fault-tolerant quantum machines are widely available, and in the meantime, healthcare innovators must work within the constraints of small, noisy devices. This creates a risk of overhype: some worry that if early expectations aren’t managed, a backlash could occur if quantum benefits take longer to materialize.
Beyond the hardware, there are regulatory and ethical hurdles. Healthcare is a highly regulated domain (for good reason: patient safety is paramount), and any quantum-derived tool will need rigorous validation. How do we verify that a quantum algorithm’s recommendation for a cancer treatment is correct or safe? If a quantum AI finds a pattern linking certain gene combinations to a disease, regulators will ask for evidence just as they would with any new diagnostic test. Current regulatory frameworks aren’t fully equipped for quantum tech. They may need updating to account for the probabilistic nature of quantum computing outputs and the difficulty in interpreting how a quantum algorithm arrives at a result. Ethically, there are concerns about data privacy and consent. If quantum computers enable analysis of combined datasets at a scale never done before (e.g. national health records plus genomic databases), ensuring patients’ privacy and proper consent for such analysis is critical. There’s also the risk of bias or unintended consequences in quantum-driven decisions – just as with AI, if the data fed into a quantum algorithm is biased or unrepresentative, the results could perpetuate inequities. And of course, as discussed, security concerns loom large: the threat of quantum computing breaking encryption could undermine public trust in health IT systems if not preemptively addressed. All these factors contribute to a cautious approach.
Another practical barrier to adoption is cost and access. Quantum computers are currently expensive to build and operate (with dilution refrigerators, specialized facilities, etc.), so initially only well-funded centers and companies can experiment with them. If the benefits of quantum computing are not evenly distributed, we risk creating a divide where only elite institutions get the advantages – a scenario policymakers and healthcare leaders are keen to avoid. Finally, there is a knowledge gap risk: quantum computing sits at the intersection of physics, computer science, and biology. Bridging these fields requires interdisciplinary experts, who are in short supply. If healthcare organizations don’t invest in training or hiring quantum-proficient talent, they may struggle to even evaluate vendor claims or research findings in this space, leading to slow uptake or missteps. In summary, while quantum computing holds great promise, it faces a gauntlet of challenges on the road to healthcare integration: immature technology, hefty resource requirements, unclear regulatory pathways, data governance questions, and human factors. Addressing these issues will require concerted effort – advancing the science to improve hardware, crafting new guidelines and standards, and educating stakeholders. The good news is that these challenges are recognized, and work is underway to overcome them. By navigating these hurdles carefully, the healthcare sector can mitigate risks and ensure that when quantum solutions do arrive, they are safe, equitable, and truly beneficial.
Conclusion
Quantum computing has the potential to reshape global healthcare and medical research in the coming decades. From our current vantage point, we can see glimmers of its future impact: prototype quantum algorithms already accelerating drug discovery, early collaborations bringing quantum hardware into hospital research labs, and quantum-inspired methods optimizing healthcare operations in ways that improve patient care. As the technology evolves from today’s nascent systems to tomorrow’s fault-tolerant quantum computers, the scale of disruption and advancement will only grow. Imagine diagnosing diseases before symptoms appear by crunching unimaginable data in seconds, or tailoring cures by simulating molecular interactions inside a patient’s cells with perfect accuracy. These possibilities, while ambitious, are what drive the intense interest in quantum healthcare. Realizing them will require continued innovation and careful implementation – scientists, clinicians, and regulators working hand in hand to validate new quantum tools and integrate them responsibly into practice. The coming quantum era will demand adaptation, but it also promises to unlock solutions to medical puzzles that have long stymied researchers. As Cleveland Clinic’s CEO aptly put it, this technology “holds tremendous promise in revolutionizing healthcare and expediting progress toward new cures and solutions for patients.” In the end, if we prepare adequately and address the challenges, quantum computing could usher in a healthier future – one where computing is no longer the limiting factor in saving lives and improving well-being. The next frontier of medicine may very well be defined by qubits, and the journey toward that future is already underway.