Quantum Computing Modalities: DNA-Based QIP
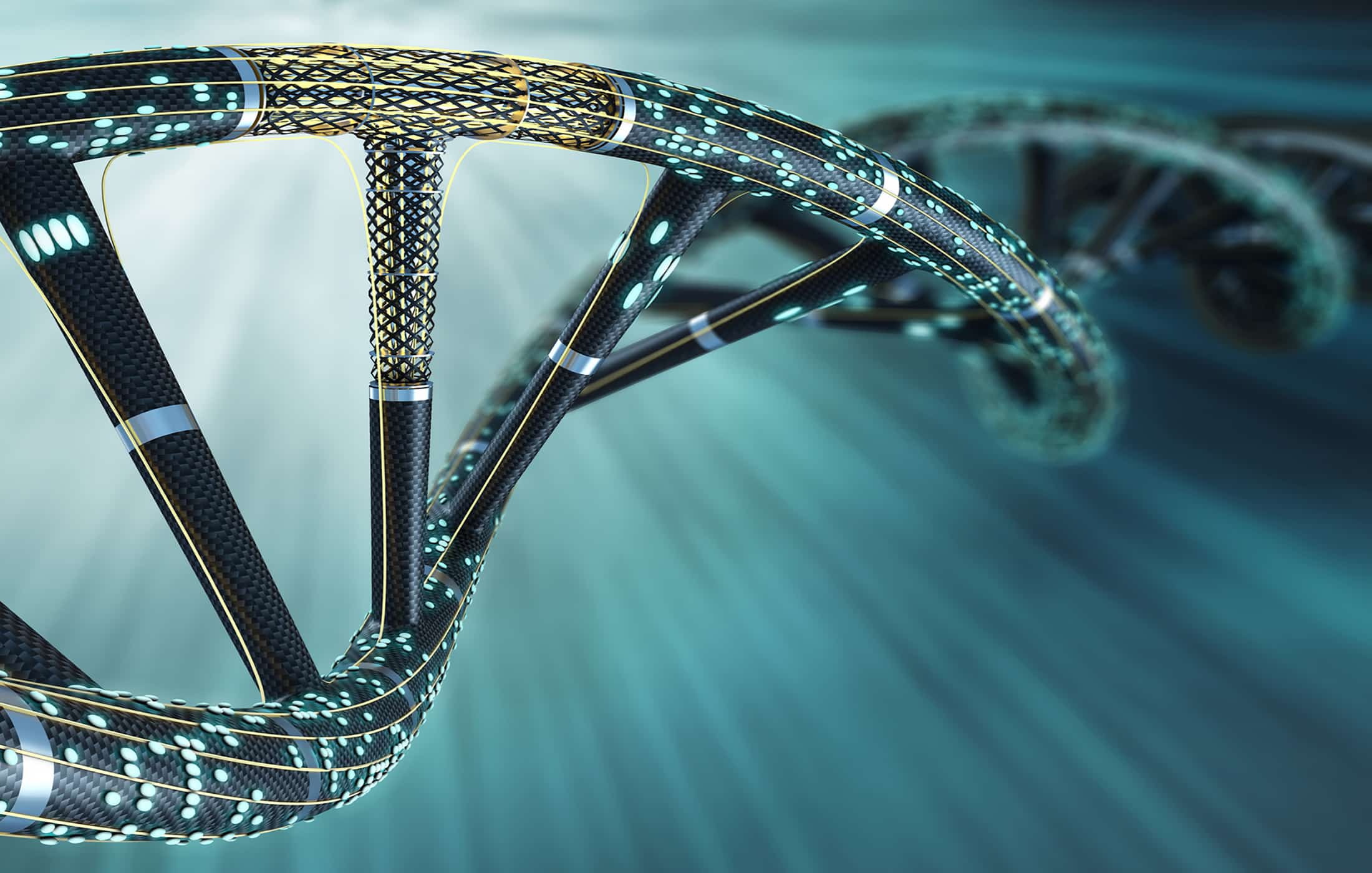
Table of Contents
(For other quantum computing modalities and architectures, see Taxonomy of Quantum Computing: Modalities & Architectures)
What It Is
DNA-based quantum information processing envisions using DNA – the molecule of life – in roles within a quantum computer. This could mean DNA acting as qubits, facilitating quantum interactions, or serving as a structural scaffold for other qubits. It’s an intersection of quantum technology with biotechnology and nanotechnology.
There are several angles to consider:
- DNA as Qubits: DNA has specific quantum aspects (like the electrons in the base pairs, or the spin of nuclei in the bases). Some researchers have speculated that the two complementary bases in a pair (A-T or C-G) could form a two-level quantum system that might be manipulated. For instance, the hydrogen bonds between base pairs could tunnel between configurations (there’s a phenomenon of proton tunneling causing A* (tautomer) pairing with C occasionally). One could imagine encoding 0 and 1 in two conformations of a base pair and using quantum tunneling as operations. A bold proposal by Riera et al. (2021) treated each A-T or C-G pair as a Josephson-like junction where the hydrogen bonds allow a shared proton to quantum tunnel, acting like a phase qubit. They suggested DNA base pairs could behave as superconducting paired elements at very low temperature, which is highly speculative and not experimentally shown.
- Nuclear Spins in DNA: Every atom in DNA has nuclear spins. Notably, the phosphorus atom in the backbone is spin-1/2 (for the dominant isotope P-31) and could serve as a qubit with relatively long coherence (phosphorus nuclear spins in a solid lattice can have long T1 and T2). Carbon-13 if present (1% naturally) in the bases is spin-1/2. One can envision using these nuclear spins as qubits and the DNA as a built-in register. NMR techniques could address these spins. In fact, liquid-state NMR quantum computing (demonstrated with small molecules in the late 90s) could in principle be done with DNA strands in solution – e.g., a 3-base-pair DNA with certain isotope labeling could be a 6-qubit NMR quantum processor. However, NMR QCs are not scalable and have issues with ensemble measurement and mixed states.
- DNA Origami for Qubit Positioning: DNA can be programmed to fold into precise 2D/3D shapes (DNA origami). Researchers are using this to arrange other quantum components. For example, one can attach quantum dots or nitrogen-vacancy centers or silicon vacancies to specific points on a DNA origami scaffold. DNA origami has achieved placement accuracy of a few nanometers. This could create a small quantum network (like multiple NV centers positioned for optical coupling, or quantum dot arrays for photonic circuits). Qubit connectivity and scaling might be improved by DNA scaffolds: instead of nanolithography, one could “grow” a circuit. For instance, a recent work used DNA origami to arrange fluorescent molecules in a nanocavity with precise spacing, effectively controlling light-matter interaction relevant to quantum devices.
- DNA-templated electron spin chains: Some propose to use DNA as a template to line up electron spins or superconducting grains. DNA is electrically a semi-conductor (with some ability to transport electrons under certain conditions). There have been experiments on DNA conduction (which show it’s not a great conductor, but maybe can hold hopping electrons). One might imagine threading electrons or polarons along a DNA to create a one-dimensional quantum register.
- Bio-quantum interfaces: Another idea is to connect DNA computing (classical) with quantum computing, perhaps using DNA to encode classical data that a quantum computer will read or vice versa.
Key Academic Papers
- A theoretical work by Artemov et al. claimed “DNA is a natural quantum computer” and talked about base pairs as Josephson junctions. It’s not mainstream but shows the idea.
- Krzysztof Petrovsky and others have papers on DNA as a quantum information channel and entanglement in DNA, but often these are speculative or even fringe.
- The concept of using DNA to assist quantum computing practically is seen in Stephan Woerner at IBM and others in a 2012 paper where they used DNA origami to arrange carbon nanotubes for electronics (not quantum, but similar principle). The idea to use DNA for arranging NV centers was explored by D-Wave researchers in a patent on “scalable QC via self-assembly”.
- Lloyd’s group (Seth Lloyd) has considered algorithms or parallels between quantum computing and biological processes. In a sense, his algorithm for solving linear equations or doing quantum walks have been compared to processes in biology (like exciton transport).
- The QIS community has occasional workshops on “bio-inspired quantum tech” – not much published output yet beyond conceptual proposals.
Comparison To Other Modalities
DNA-based QIP is not a fully separate model like circuits vs MBQC; rather, it’s a potential physical implementation or augmentation of quantum processors. It could work in tandem with other modalities: e.g., you might have a hybrid device where superconducting qubits are wired together by DNA scaffolds with quantum dots acting as couplers (photonic or spin mediators). In terms of information processing capabilities, any DNA qubit would follow the rules of quantum mechanics like any qubit, so presumably they could do what other qubits do, if coherence and gate control are achieved.
However, DNA stands out by:
- Molecular scale and self-assembly: It offers extremely fine feature sizes (2 nm helix diameter) and bottom-up assembly, whereas most quantum computing to date is top-down (fabrication or placing components by machines). That could allow scaling to very high qubit counts cheaply if we figure out how to incorporate active qubits into DNA structures reproducibly.
- Biocompatibility: If one dreams of quantum sensors in biological environments (say qubits used to probe inside a living cell), DNA could be a biocompatible part of that system. E.g., one could attach a qubit to a DNA that targets a specific cell structure for quantum sensing (some quantum biosensing experiments do attach nanodiamonds to DNA or proteins).
- In contrast to purely solid-state or optical systems, DNA-based approaches might leverage chemical processes for configuration (like DNA hybridization can bring qubits together or apart in solution, acting as a reconfigurable quantum gate mechanism possibly).
Advantages
- Precision Nanoscale Structure: DNA origami can position objects with nanometer precision in complex 2D/3D patterns. This is extremely useful in quantum computing where alignment of components (like resonators, waveguides, and qubits) is critical. It’s like having a programmable breadboard at the scale of macromolecules. For instance, a DNA-based array of quantum dots could allow controlled exchange interactions (for spin qubits) or photonic coupling paths.
- Mass Production by Self-Assembly: Once you have a DNA sequence that folds into a desired quantum circuit layout, you can produce billions of copies by just running a biochemical reaction (mixing the strands). This could drastically reduce cost per device if it can incorporate the quantum components. In principle, one could “grow” qubit arrays instead of nanofabricating each one.
- Hybridization as Computation: DNA molecules can recognize complementary sequences and bind (hybridize). This is a form of computation (like matching). In a quantum context, one could conceive of using DNA hybridization to bring qubits into interaction or to trigger a quantum gate. For example, two qubits attached to separate DNA strands might only come into proximity (and thus interact) when a third “bridge” DNA strand causes the two to join. This could act as a quantum controlled-operation managed by DNA inputs (though the speed of DNA binding is slow, ms to s).
- Biological Interface: Using DNA and biomolecules means one can interface quantum devices with biological systems more naturally. This is beneficial for quantum sensors for medical or environmental applications. But for computing specifically, maybe in the distant future embedding quantum processors inside living cells or organisms (for example, cellular automata with quantum elements for massively parallel computing).
- Unique Qubits: If the MDPI-like idea were real, DNA base pairs being superconducting phases, that’s a wholly new type of qubit not relying on metal Josephson junctions but on molecular bonds. DNA could theoretically have many such junctions (every base pair a junction) and you get a large qubit register in one molecule. Also, nuclear spin qubits in DNA could have super long coherence (nuclear spins are isolated from environment often; in a molecule they couple to other spins but through well-defined couplings that could be refocused via NMR techniques).
Disadvantages
- Decoherence in Wet Chemistry: DNA in its natural state is in water (unless dried). Water and the thermal vibrations at room temperature cause decoherence. If using DNA’s own quantum states, one likely needs cryogenic temperatures or to isolate them from water (dry DNA), which then loses some biological convenience. Also, DNA has electric charge (phosphate backbone is charged), meaning it’s coupled to ionic environment, which adds noise.
- Lack of Control Methods: We don’t currently have methods to address individual quantum states in DNA. How to perform a single-qubit gate on a nuclear spin in one base, without affecting others? NMR can address spins by frequency, but in a large DNA many spins have similar environment thus overlapping spectra, limiting distinguishability. For electron-based states, we would need to attach electrodes or optical reporters to DNA, which partly defeats the purpose of using DNA (since then we basically turned it into a nanowire in a circuit).
- Stability and Error in Self-Assembly: DNA origami structures aren’t perfect; they may mis-fold or break. Attaching qubits (like an NV center in a nanodiamond) to a DNA strand often yields a distribution of positions rather than a single precisely placed one due to slight flexibility or attachment site uncertainty. At small scales, even a few-nm deviation matters. Also, thermal fluctuation can cause the DNA scaffold itself to wiggle (unless fixed on a substrate). So the “precise positioning” may have some error bars. For quantum operations, jiggling of a qubit by even a nm might change coupling strength unpredictably.
- Integration: Getting a DNA-assembled array of qubits integrated with, say, microwave control lines or optical fibers is non-trivial. One might have to deposit the DNA structure onto a chip and somehow align it. There’s a material compatibility challenge: DNA exists in solution, but many quantum operations need vacuum or solid state environments.
- Computation Speed: Biological or chemical processes (like DNA movement, or even electron transfer through DNA) are typically slow relative to GHz gate rates in superconducting qubits. If we rely on chemical triggers for gates, the clock speed might be seconds or more, which is a trillion times slower than typical quantum gates. So unless the DNA’s quantum transitions are driven by external fast fields (i.e., treat it like a normal quantum chip but made of organic material), the speed is an issue.
- Research Immaturity: DNA-based QIP is at a very early stage. We have concepts and small demonstrations like placing quantum dots, but no working “DNA quantum gate” has been shown. It’s behind other modalities in development.
Cybersecurity Implications
Like biological QC, currently none specific. If DNA-based qubits became viable, they’d just be another physical platform for quantum computers – affecting timelines if they allow faster scaling or cheaper devices, but not introducing unknown algorithmic capabilities. One interesting angle is DNA cryptography: there’s classical DNA steganography and one-time pads, etc. If one had a DNA-based quantum computer, perhaps one could even embed cryptographic keys in DNA and have the quantum computer act on them only when a correct complementary DNA strand (key) is present, etc. But that’s fanciful.
One nearer-term consideration: quantum hacking of DNA sequencers. Not exactly QIP, but DNA sequencing and synthesis are computational biology tasks. If quantum computers (of any type) get good at certain kinds of searches or optimization, they might also break certain bioinformatics encryption or DNA watermarking schemes. Conversely, DNA might be used as a storage medium for quantum keys or quantum random numbers (some companies are exploring DNA data storage, which could store outputs of quantum RNGs for later use, etc.).
If DNA nanotech allows building quantum devices much smaller and cheaper, then the accessibility of quantum computing could increase – meaning more entities can have powerful quantum machines, potentially including adversaries. That broadens the threat landscape, making it even more pressing to transition to quantum-resistant cryptography widely (no longer only nation-states could have QC, but maybe companies or criminal organizations if it’s cheap enough).
Who’s Pursuing
- Academic nanotechnology groups: e.g., Hao Yan (ASU) and Lulu Qian (Caltech) are DNA nanotech experts; while they focus on classical DNA computing and nanorobots, they lay groundwork that quantum researchers could piggyback on. Some groups at Oxford and Cambridge work on DNA origami optical cavities (placing emitters).
- NIST and other national labs have some interest in molecular qubits (like using molecules that can be chemically assembled to have certain spin states – e.g., vanadium complexes as qubits that can be chemically linked).
- Quantum computing companies typically are not directly using DNA, but some collaborate with nanotech groups for packaging. E.g., IBM might look at self-assembly to place thousands of qubits on a chip, etc.
- DIA (Defense Intelligence Agency) had a report discussing utilizing organic molecules in computing, which included forecasting of DNA computing tech. They predicted some DNA-based devices in decades, though largely classical. Government foresight folks do consider long-term tech like DNA-based computing.
- No dedicated DNA-QC startup exists as of yet (unlike DNA digital storage startups or DNA computing for diagnostics). It’s mostly academic exploration.
In conclusion, DNA-based quantum information processing is a futuristic modality that merges biotech with quantum tech. It holds promise for constructing complex quantum systems by leveraging nature’s construction kit (DNA), but it faces significant challenges. If overcome, it might give us quantum computers that are literally grown rather than built, potentially transforming how we deploy computing hardware. It’s an area to watch on a 10+ year horizon, and for now it stimulates creative ideas about hybridizing fields to push computing boundaries.