Jiuzhang 3.0: China’s Photonic Quantum Computer
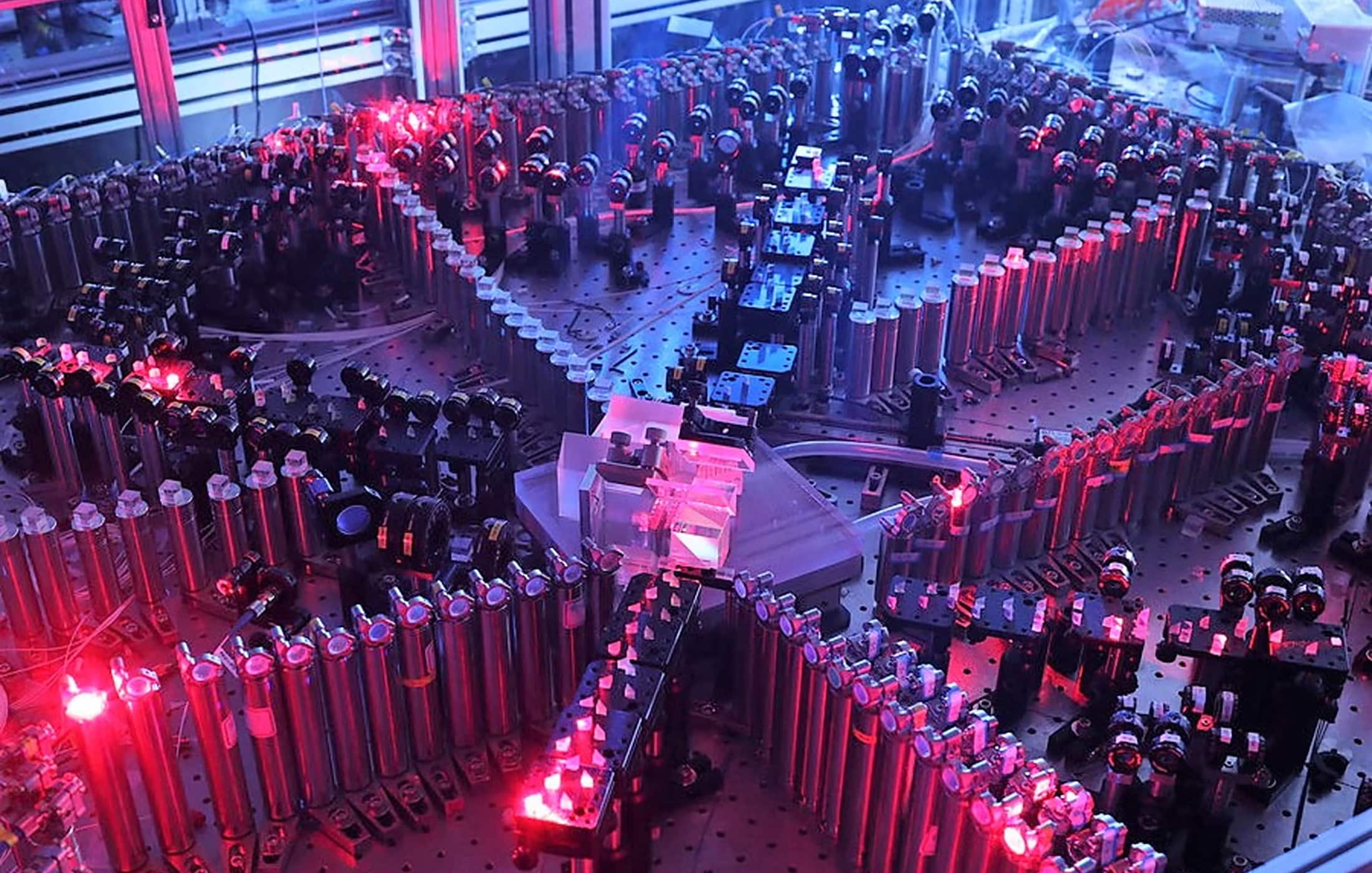
Table of Contents
Chinese researchers have announced Jiuzhang 3.0, a new photonic quantum computing prototype that set a record by detecting 255 photons in a boson sampling experiment. Unveiled in October 2023 by a team led by renowned physicist Pan Jianwei, Jiuzhang 3.0 pushes the boundaries of photonic quantum computing with a demonstration that is 10 quadrillion times faster at solving a Gaussian boson sampling problem than the fastest classical supercomputers. Pre-print of the related paper is here: “Gaussian Boson Sampling with Pseudo-Photon-Number Resolving Detectors and Quantum Computational Advantage“. This milestone firmly advances the frontier of quantum computational advantage in photonics, outpacing both the team’s earlier machines and rival systems worldwide.
A 255-Photon Quantum Advantage Demonstration
Boson sampling – specifically Gaussian boson sampling (GBS) – was the chosen benchmark task for Jiuzhang 3.0’s feat. GBS is a specialized but classically intractable problem often used to showcase quantum speedups. In essence, it involves sending many photons through a complex interferometer and sampling the outcome distribution, a task that becomes exponentially harder as more photons are involved. Jiuzhang 3.0 registered 255 photon detection events, an unprecedented scale for this experiment. Each additional photon roughly doubles the complexity of boson sampling, so moving from previous 113-photon tests to 255 photons represents an enormous leap in computational challenge. By one estimate, generating a single output sample from Jiuzhang 3.0’s distribution on the world’s top supercomputer (Frontier) would take around 600 years, whereas Jiuzhang 3.0 produces that sample in about 1.2 microseconds. In fact, the most complex outputs from Jiuzhang 3.0 would take on the order of 1010 years on Frontier to simulate exactly – effectively forever on human timescales – underscoring a clear quantum computational advantage.
This achievement vastly outclasses the team’s own earlier records and those of competitors. Jiuzhang 1.0, unveiled in late 2020, first put China on the quantum advantage map with 76-photon boson sampling. It made China the second country (after the U.S.) to claim “quantum supremacy,” performing its specialized calculation far faster than any classical computer could. The follow-up Jiuzhang 2.0 in 2021 pushed the photon count to 113 and further increased the computational speedup. Jiuzhang 3.0’s 255-photon result now leapfrogs these, running GBS 1,000,000× faster than Jiuzhang 2.0 by the team’s estimates. To put global context around these numbers, the previous photonic quantum computing record was held by Borealis, a photonic machine from Canada’s Xanadu, which reached 219 detected photons in a 2022 GBS experiment. Borealis could sample distributions in about 36 µs that would require an estimated 9,000 years on a classical computer – impressive in its own right, but Jiuzhang 3.0 has now raised the bar further in both photon count and speed.
From Jiuzhang 1.0 to 3.0: What’s New and How It Compares
Each iteration of the Jiuzhang series marked a significant step forward in photonic quantum computing. The original Jiuzhang 1.0 demonstrated the viability of using squeezed-light sources and linear optics to achieve a quantum advantage, but it was essentially a proof of concept device designed for one task (GBS). Jiuzhang 2.0 improved on this with more photons and a 144-mode optical circuit, making the quantum experiment’s state space even larger and further out of reach for brute-force classical simulation. Notably, Jiuzhang 2.0’s 113-photon experiment was described as performing GBS septillion times faster than the best supercomputers at the time, firmly reinforcing the quantum advantage first claimed by Jiuzhang 1.0.
Jiuzhang 3.0 builds on its predecessors with a combination of scale and new technology. It still relies on a photonic approach – using pulses of laser-generated squeezed light as the source of quantum states, which are then interfered in a large optical network – but introduces a novel detection scheme to handle the torrent of photons at the output. In earlier versions, the detector array was a bottleneck: standard single-photon detectors could “click” to register a photon’s arrival but could not effectively distinguish multiple photons arriving simultaneously at the same output mode. For Jiuzhang 3.0, Pan’s team developed a “pseudo-photon-number-resolving” detection system to overcome this limitation. As team member Lu Chaoyang explained, they implemented a superconducting nanowire single-photon detector (SNSPD) array with a fiber loop delay network, which demultiplexes photons into time bins before detection. In practice, this means that if multiple photons emerge at one detector port at the same time, the fiber loops stagger their arrival times so the nanowire detectors can register them as separate clicks. This innovation greatly increased the count of photons that can be detected in a single experimental run, enabling the leap to 255 detected photons. Combined with an ultralow-loss optical circuit and high-purity light sources, Jiuzhang 3.0 was able to significantly boost the complexity of the boson sampling task it tackles.
Beyond the hardware upgrades, the research team also took care to verify that Jiuzhang 3.0’s results indeed reflect quantum computational advantage and not some subtle classical shortcut. They performed rigorous statistical validation of the output data, including Bayesian hypothesis tests and correlation analyses, to rule out any “spoofing” by classical algorithms or imperfections. In other words, the randomness and correlations in the 255-photon samples were checked to ensure they match genuine quantum predictions and can’t be efficiently mimicked by known classical methods. This adds confidence that Jiuzhang 3.0’s experiment satisfies the criteria for quantum advantage in the same spirit as Google’s 53-qubit Sycamore processor did for random circuit sampling in 2019, and as earlier photonic experiments did for boson sampling.
Why It Matters in the Quantum Computing Landscape
Jiuzhang 3.0’s accomplishment is significant for quantum computing on multiple levels. First, it demonstrates the continued scalability of photonic quantum computing. Photonic systems had long promised certain advantages – such as low noise at room temperature and high communication bandwidth – but scaling them to a regime of quantum advantage was a major experimental challenge. The Jiuzhang series, alongside other photonic efforts like Xanadu’s Borealis, show that optical approaches can keep pace in the race for quantum computational power, alongside superconducting qubit platforms (like those from Google and IBM) and atomic qubit systems. With 255-photon entangled states now achievable, photonic processors have reached problem sizes well beyond what classical computing can brute-force, highlighting photonics as a viable quantum computing pathway.
Moreover, the technical breakthroughs in Jiuzhang 3.0 address key bottlenecks for the field. The pseudo-number-resolving detector technique is an advance that could be useful in any optical quantum computing setup, potentially aiding future photonic machines that require handling many photons or even enabling error-corrected photonic qubits. By drastically increasing the number of detected photons, Jiuzhang 3.0 also pushes experimental quantum physics into a new regime, allowing researchers to study richer quantum interference phenomena and test the limits of computational complexity. Each such leap tends to spur improvements in classical algorithms as well – indeed, the team noted that the contest between quantum hardware and classical simulation is a “long-term competition” where classical methods will also improve. But for now, Jiuzhang 3.0 widens the gap, delivering results in microseconds that would take classical computers billions of years.
It’s important to note that boson sampling machines like Jiuzhang 3.0 are specialized, not general-purpose computers. They can’t run arbitrary algorithms or solve an array of practical problems; they are built to tackle one specific mathematical sampling task extremely fast. As such, this breakthrough is a demonstration of quantum advantage rather than a step directly toward a universal quantum computer. Even Pan Jianwei’s team acknowledges that we are “still a long way” from general-purpose quantum computers, which would require error correction across millions of qubits. Nonetheless, achieving quantum advantage at larger scales is a crucial benchmark for the field. It validates that quantum mechanics continues to confer exponential computing power increases as systems grow, and it builds confidence that investing in scaling up quantum machines is scientifically fruitful.
In the broader quantum computing landscape, Jiuzhang 3.0 reinforces that there are multiple technology paths racing forward. While industry heavyweights focus on superconducting and trapped-ion qubits, the success of a photonic platform at this level suggests that photons could play a major role in the quantum computers of the future – whether as processors themselves or as interconnects in hybrid systems. Jiuzhang 3.0’s 255-photon milestone is not an end-point but a stepping stone. It spurs on both quantum hardware developers and classical algorithm designers, and it underscores how quickly the frontier of “impossible for classical” is being pushed outward. By decisively outperforming classical computation in its chosen task, Jiuzhang 3.0 marks another leap in quantum computing’s advance, offering a glimpse of the extraordinary computing regimes that quantum machines are beginning to unlock.